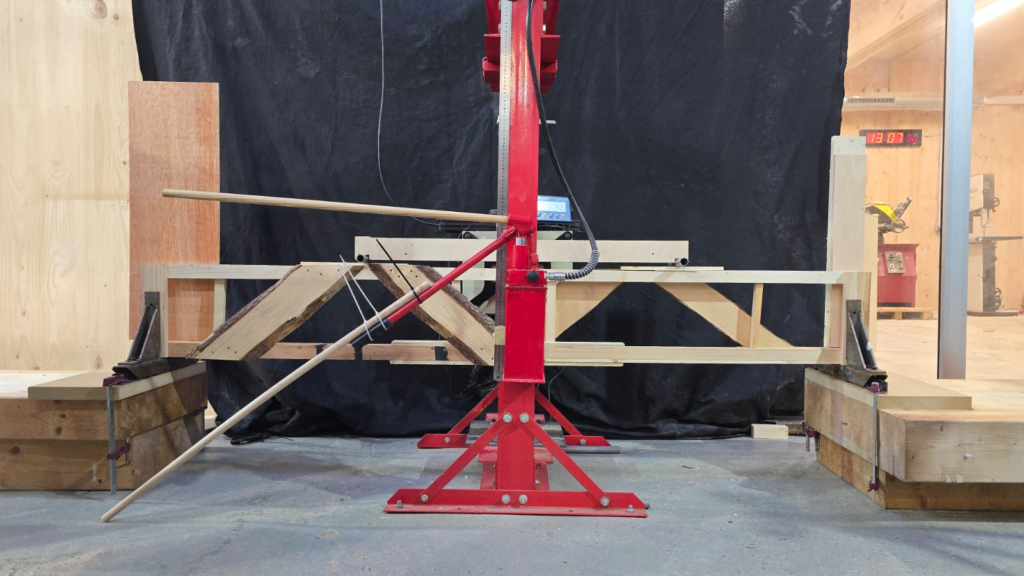
Stability Split project explores sustainable construction using scavenged materials and recycled wood for a structural beam. In the first phase, a case study of the Google headquarters in London was analyzed using Karamba software to explore structural efficiency and redesign its system. Two design options—arched and stacked—were proposed as alternatives to improve structural performance and space utilization. In the second phase, a beam made from recycled materials is tested for its strength and load-bearing capacity, with the goal of validating the potential of recycled materials in construction and inspiring sustainable practices.
Case Study
Google Bay View; BIG + Heatherwick Studios
- Original Structural System
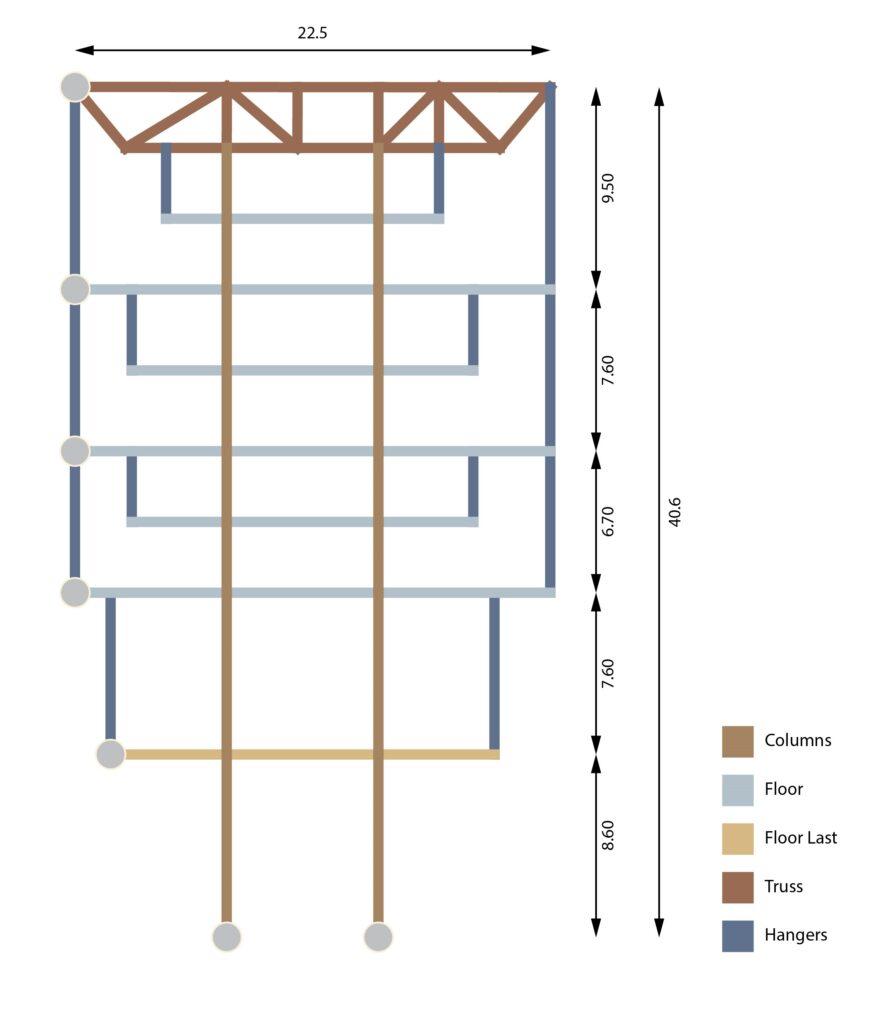
The original design uses a truss system to hang the floors in tension, while using the columns to transfer down the weight of the compressive force creating an up and down movement of force loads.
2. Iteration 1: Arched Structural System
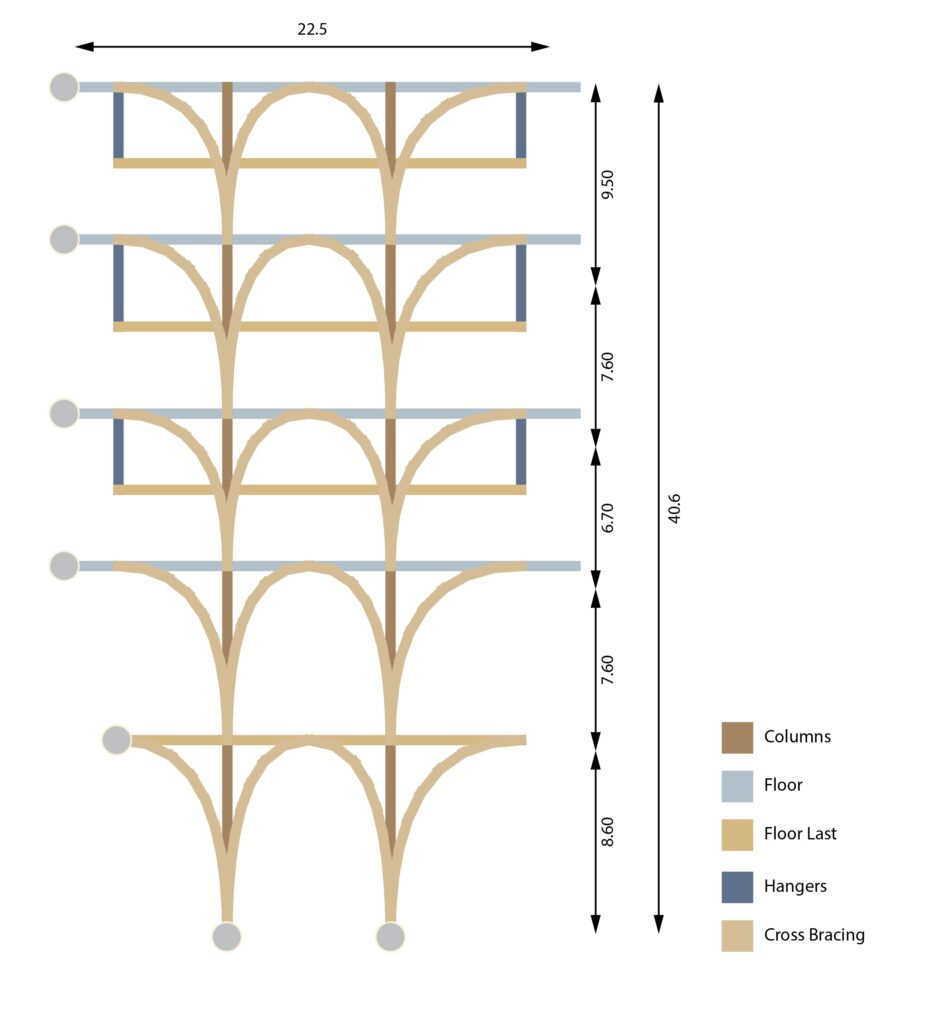
By eliminating the truss system, we proposed an arched based structural system to maximize stability, especially in the lateral load due to the load distribution of arch design. This has the lowest utilization and gives us a futural design system allowing us to maximize live load.
3. Iteration 2: Stacked Structural System
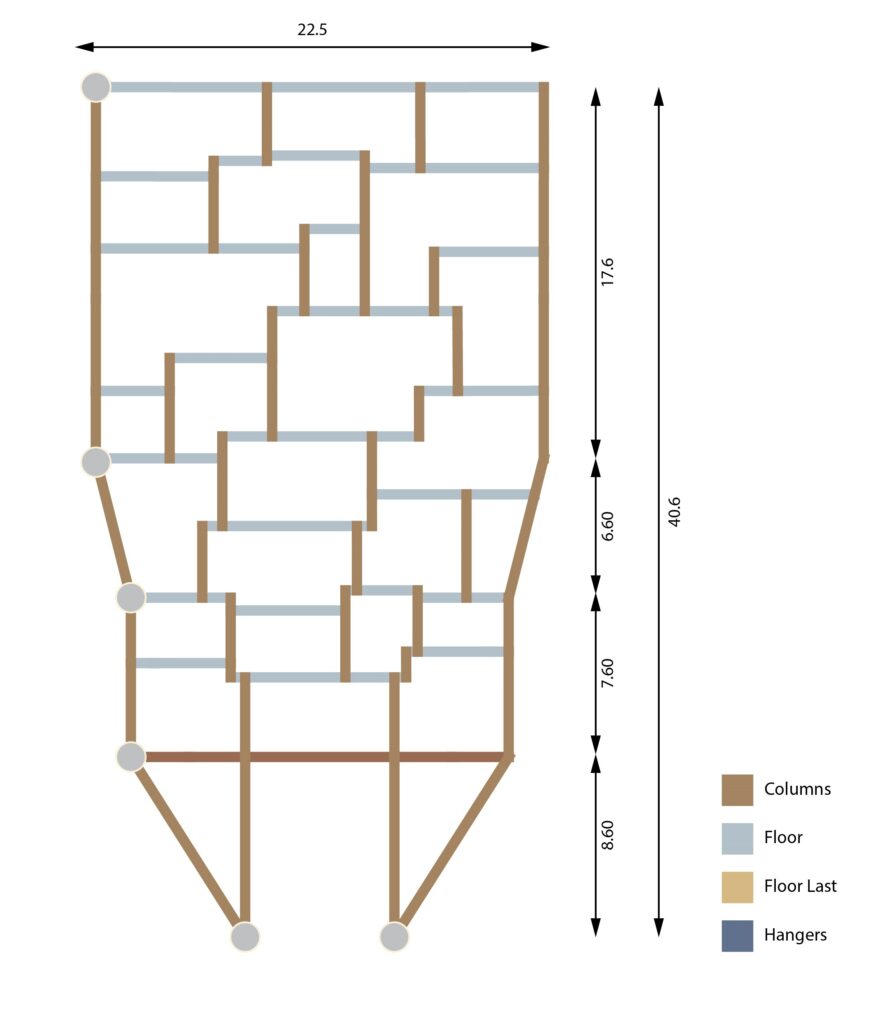
Again by removing the truss and opting for a stacked infill, we can use maximum utilization of the internal space while opting for maximum stabilization, creating a honeycomb like structure that keeps the building in a higher state of utilization.
Utilization comparison
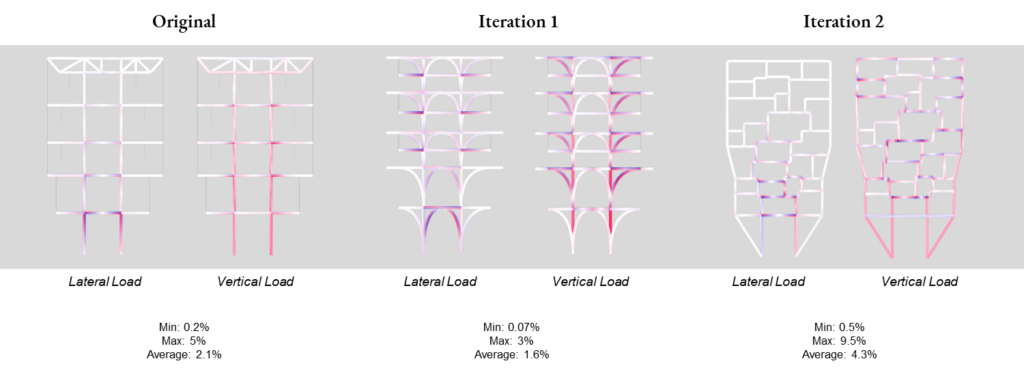
Comparing out the Utilization models through Karamba we can analyze the performance from a perspective of min, max, and average of the three iterations. Iteration 1 has the lowest percentage utilization giving it the best futurability performance, followed by the original and then by our stacked structural design.
Displacement comparison
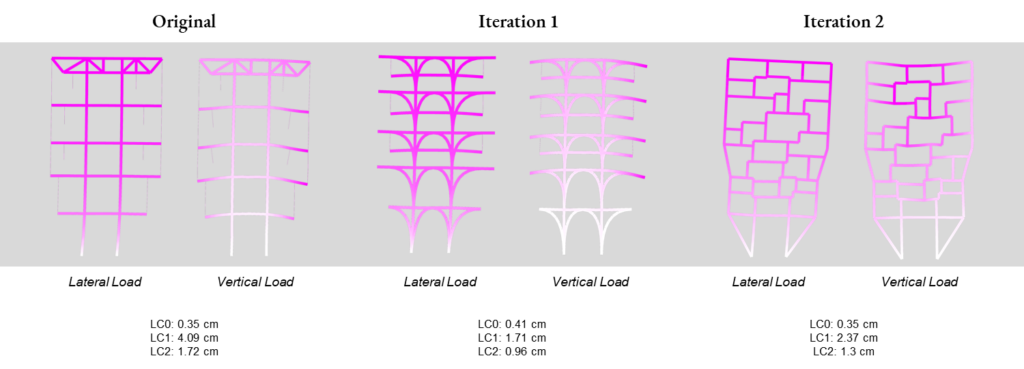
Using Karamba we can differentiate the displacement for each load we apply to the iterations and provide a max change in displacement. Iteration 1 performs the best laterally using arches as a successful way to transfer these lateral loads. Iteration 2 also successfully reduced deformation with its more honeycomb like structure allowing the whole building to resist the lateral forces. The original design has the weakest resistance to deformation due to lack of cross bracing.
Bending in Y Axis (My) comparison
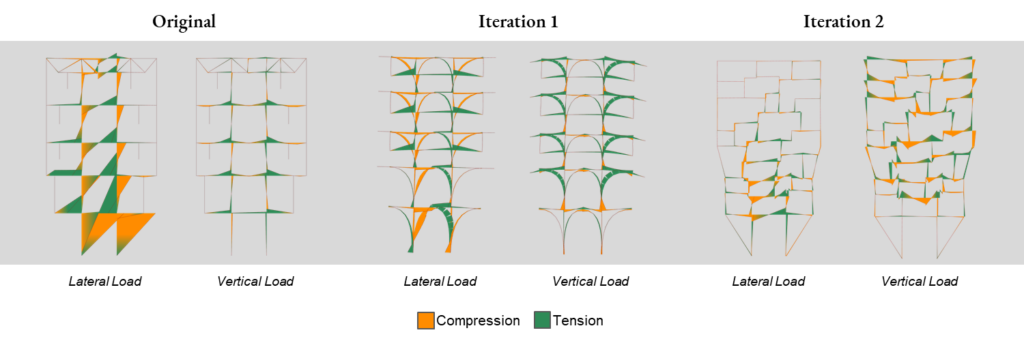
Viewing the My comparison allows us to further identify the visual load comparisons from the utilization and displacement, illustrating how the beams and columns cope with the forces distributed along the y axis. We can see how the bends in tension and compression work along the beams to columns.
Nx Load comparison
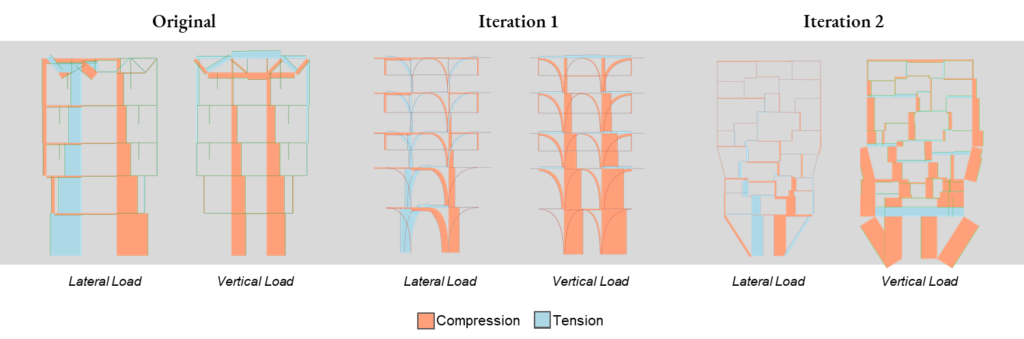
Visualizing in the Nx provides information on the transfer of loads across our columns and beams where the highest compressions and tensions are located.
Beam Design
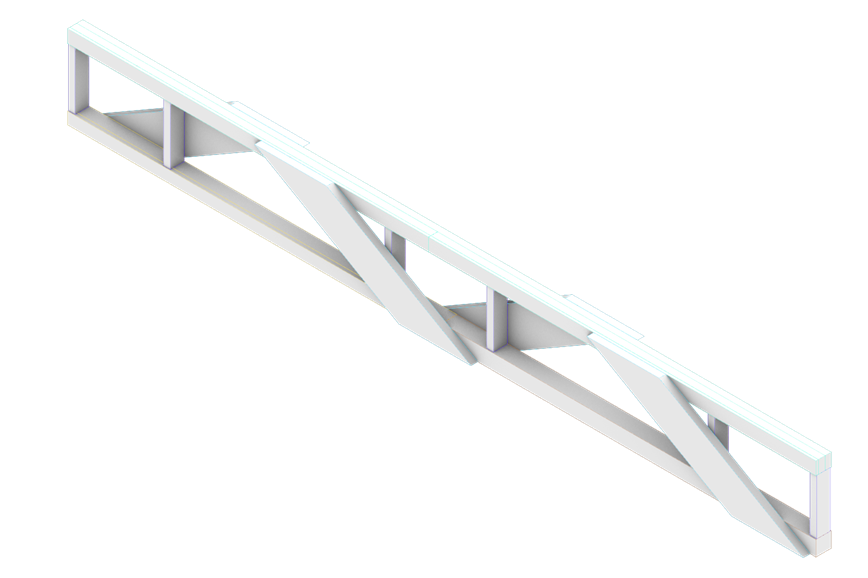
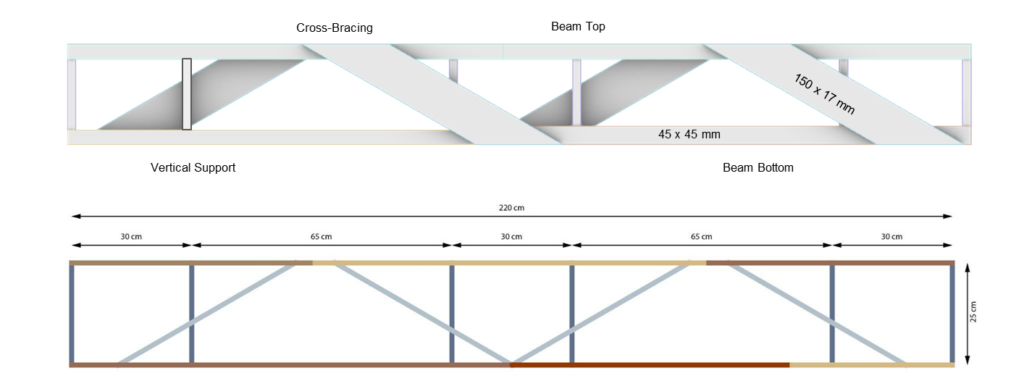
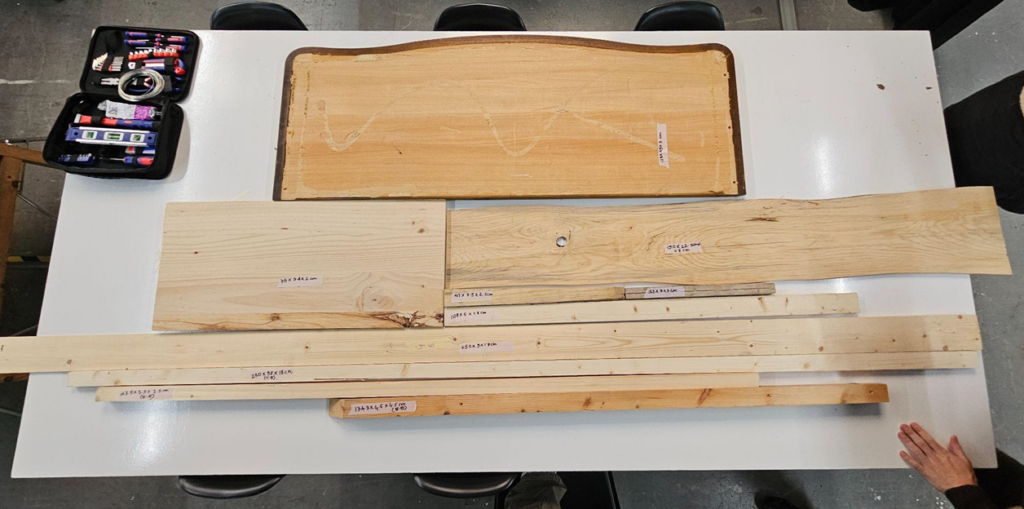
Beam Iterations
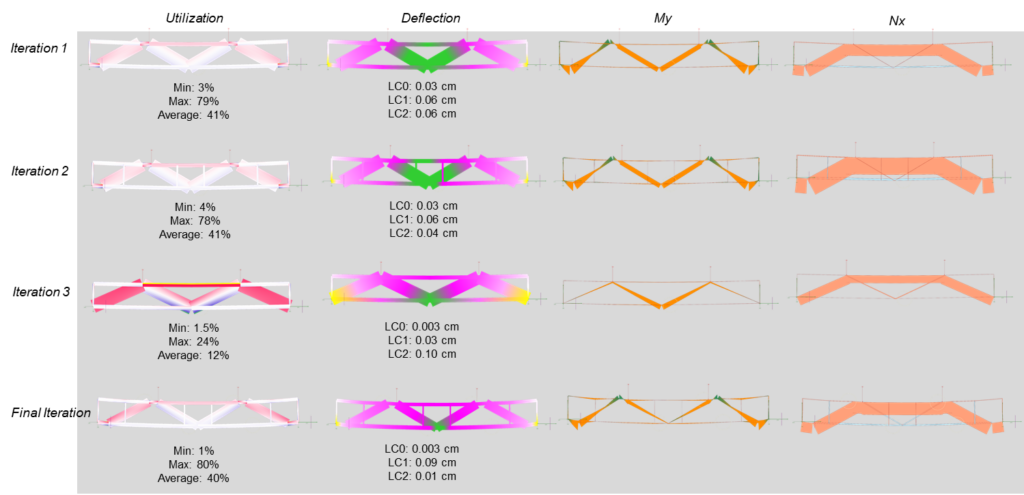
In the initial iteration, cross braces overlapped with the splice, but structural analysis revealed potential failure points. Iteration 2 repositioned vertical supports to address these weaknesses, reducing deflection though without significant change in utilization. In the final iteration, braces overlapped with the end vertical supports, and vertical supports were further refined to maximize overall strength and stability. This resulted in a final design with significantly improved performance and minimal displacement.
Fabrication
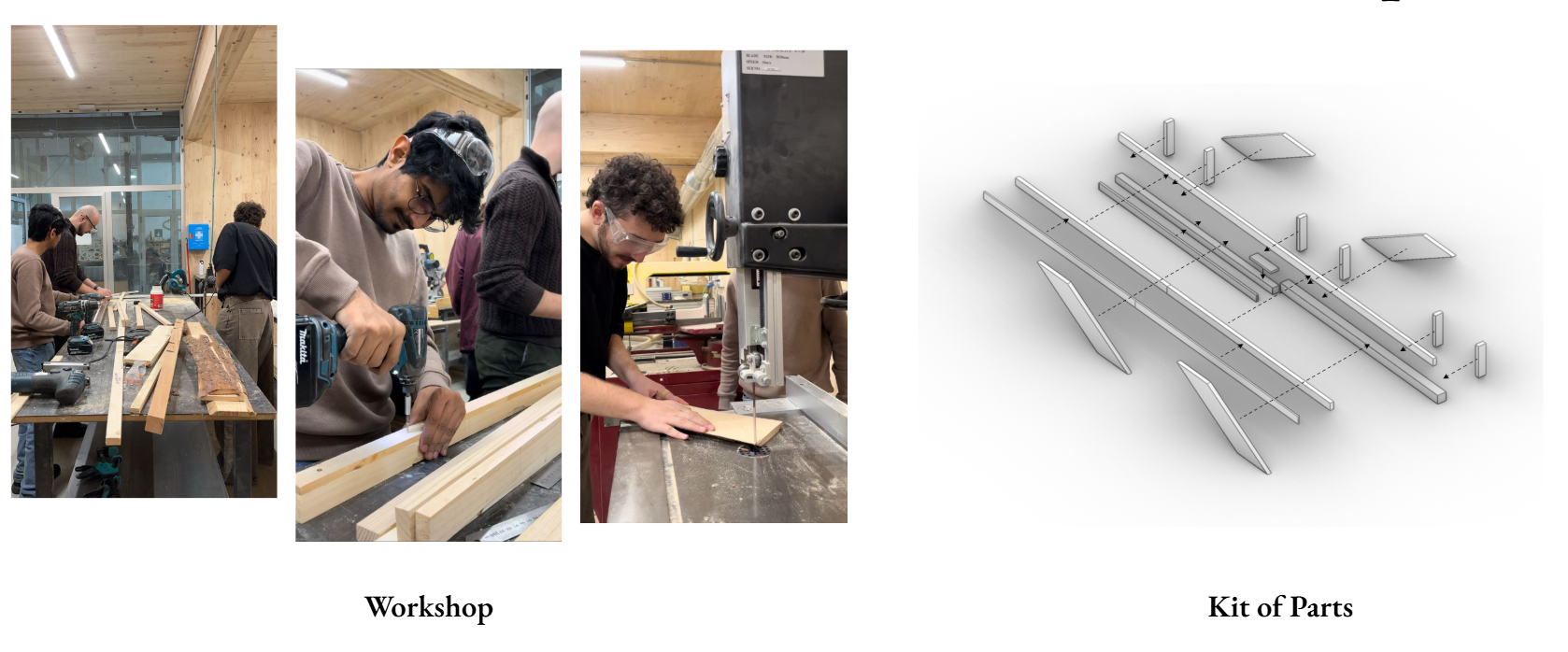
Strength Testing
Displacement in Beam
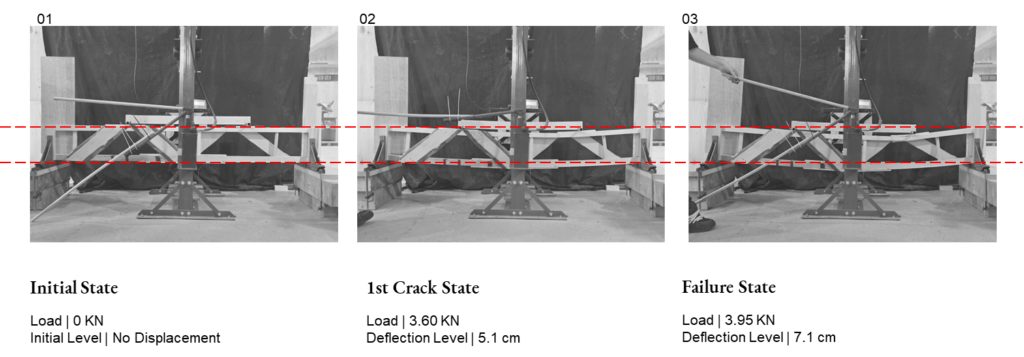
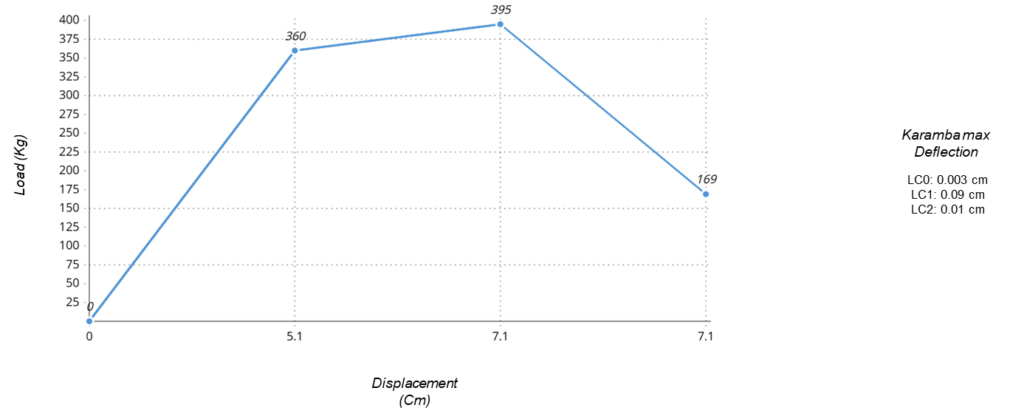
When testing the beam’s load-bearing capacity and deformation characteristics, The first crack was heard under a load of 360 kg, accompanied by a displacement of 5.1 cm. Ultimate failure occurred at 395 kg, with a maximum displacement of 7.1 cm. After releasing the load, the beam exhibited permanent deformation, retaining a displacement of 4.1 cm. These results highlight the beam’s structural limits and the permanent effects of overloading.
Failure in Beam
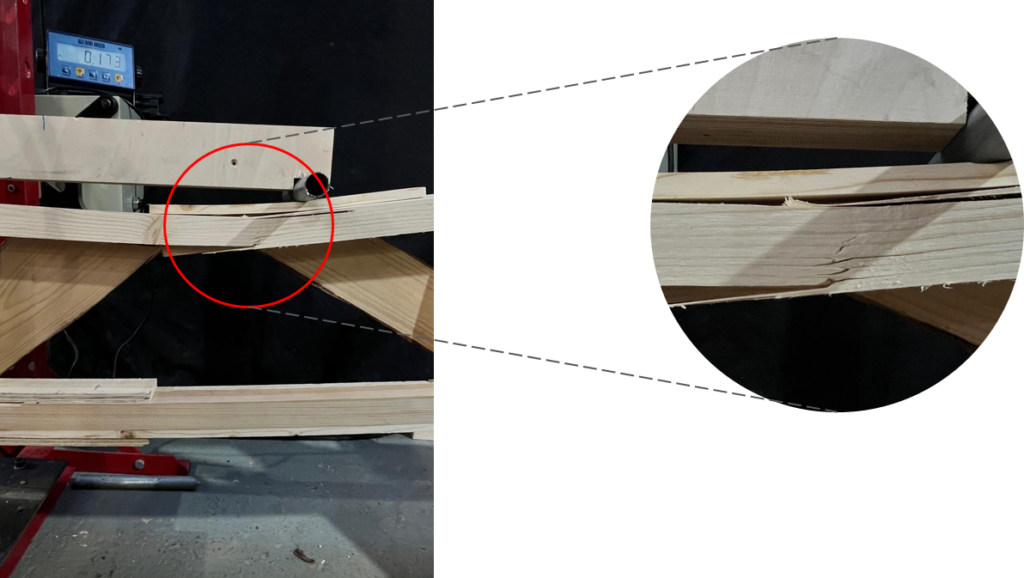
Failure Mode: Shear Failure at splice due to misplaced cross-bracing
Improvements: Attach cross-bracing element along both sides of the splice, to ensure they are equally supported
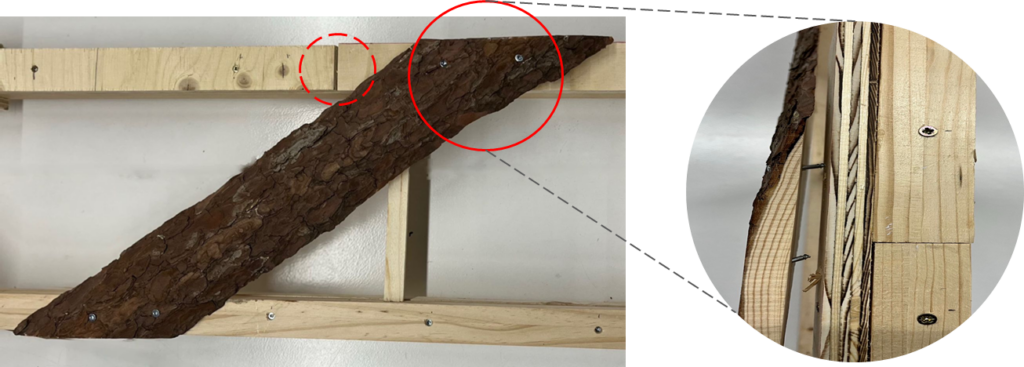
Failure of the cross-bracing: The nails bent at a 45 degree angle under the pressure. The diagonal strut would have been much more optimized away from the splice.
2 Point load Vs. Snow load
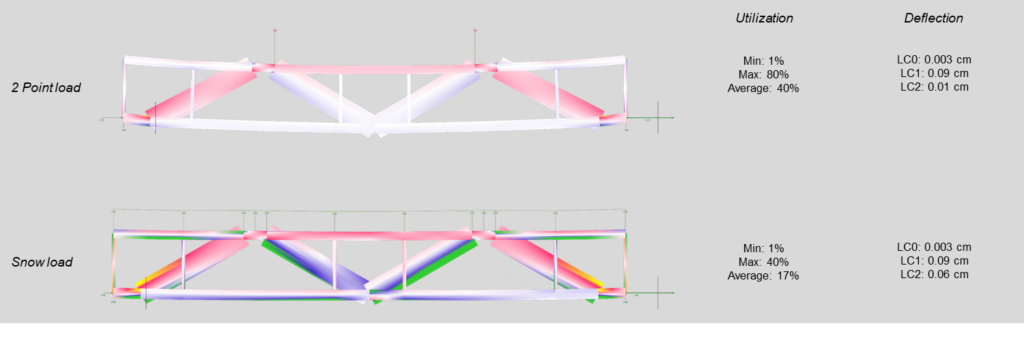
Embodied Carbon – Beam
Reusability vs Embodied Carbon
By using recycled timber that we located ourselves, we remove the two other aspects involved in embodied carbon; transport and manufacturing. We now only need to calculate the embodied carbon of our beam from the carbon stored within our material choice. Timber being one of the lowest embodied carbon with a value of 0.236KGC02e we can multiply this by the weight of our beam to find the equivalent embodied carbon within. This gave us an embodied carbon value of 1.69 C02KG for 7.1 KG mass (karamba analysis)
Calculation with Grasshopper Script (Karamba)
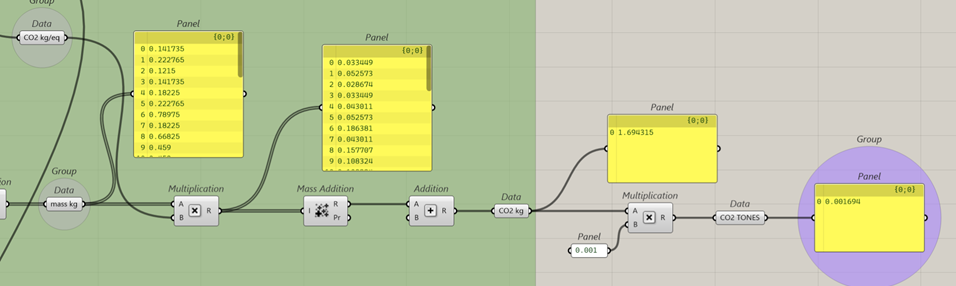
Calculation for Prototype

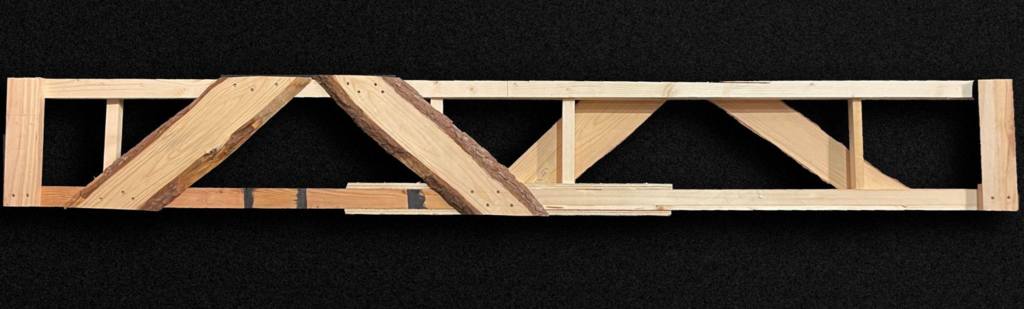
Our explorations throughout the design, fabrication, and analysis of Stability Split highlights the immense potential of integrating technology with sustainable practices to revolutionize material reuse in construction. If a few scavenged wooden pieces can be assembled to carry 395 kg (i.e. 58 times its own weight), imagine the opportunities in reusing entire building foundations or repurposing structural components of existing buildings. Tools like Karamba empower designers by visualizing structural integrity, enabling precise calculations of member utilization. This ensures efficient resource management, minimizes waste, and optimizes material allocation by identifying when excess materials are unnecessary. Such innovations redefine how we approach sustainability, moving beyond simply reducing emissions to actively reimagining our relationship with existing resources. By embracing this integration of technology and recycled materials, we unlock a future where entire structures can be repurposed, contributing to a circular construction economy. Structural design, therefore, becomes not just about solving engineering challenges but also about addressing the climate emergency through innovative, adaptive, and regenerative practices.