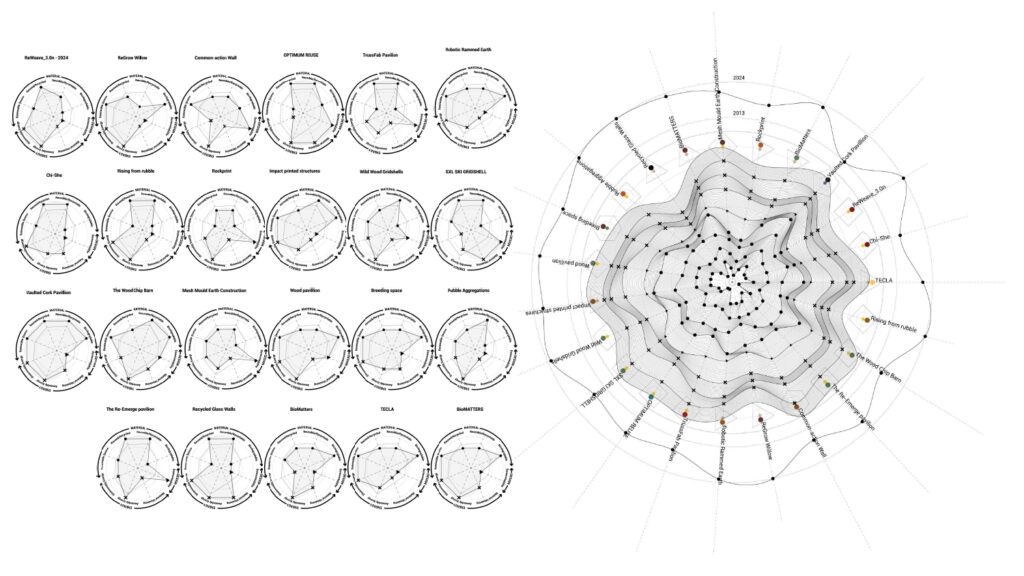
The construction industry has long been a major contributor to global resource consumption and waste production. In response to these challenges, circularity in construction has emerged as a transformative approach aimed at reducing environmental impacts, conserving resources, and promoting sustainability. This blog explores the core parameters of circularity in construction—materials, design, and energy consumption—and provides an analysis of notable case studies to illustrate the application of these principles.
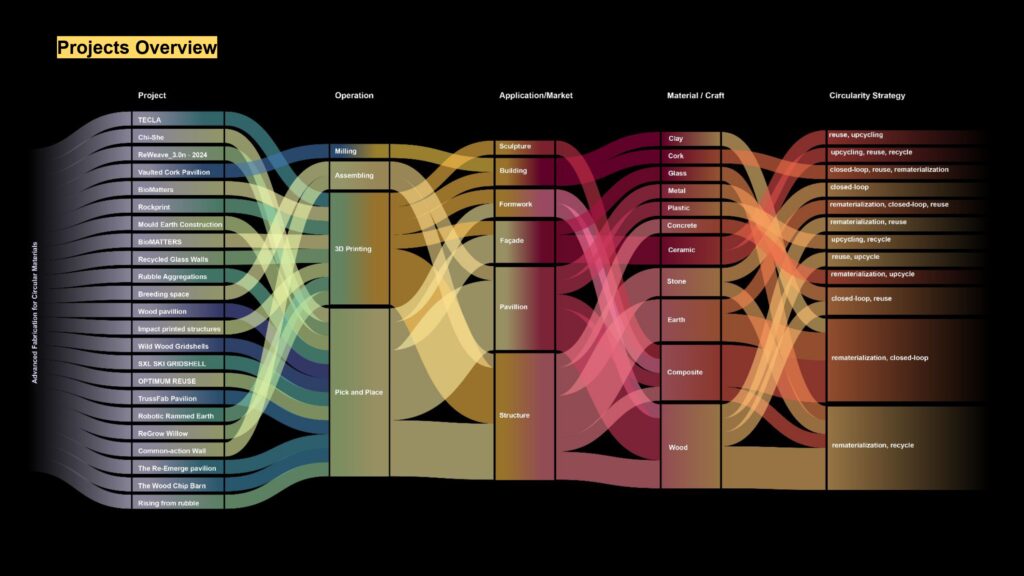
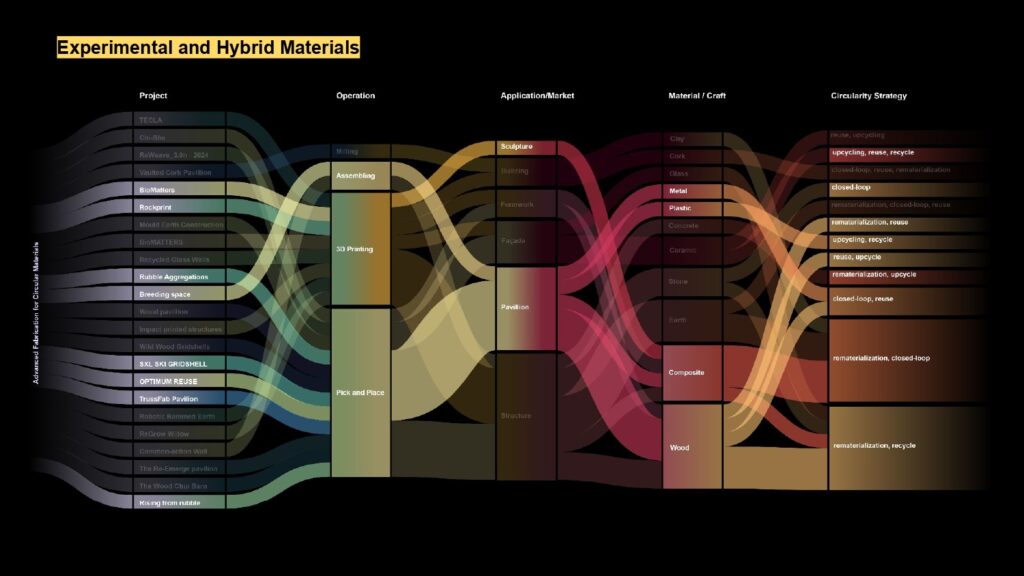
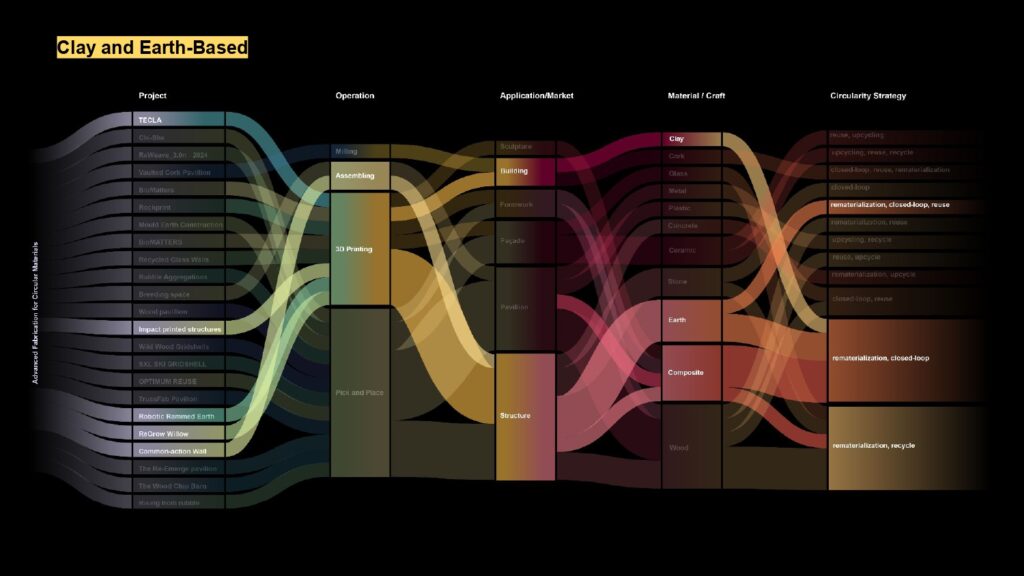
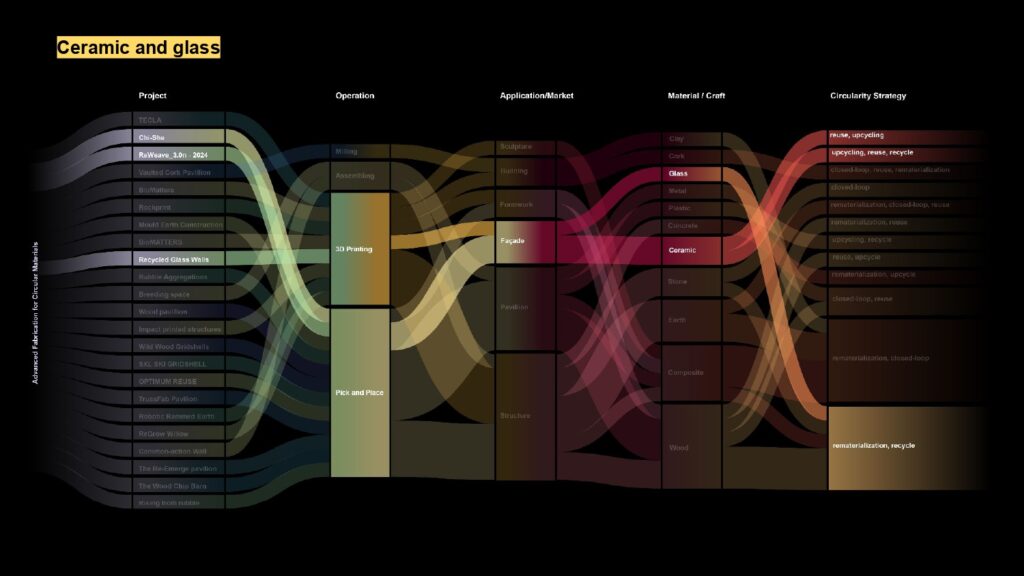
Materials
Renewable Sources
Materials derived from regenerating resources, such as natural clay or timber, minimize dependence on finite resources. For instance, the Vaulted Cork Pavilion demonstrates the use of cork, a fully renewable resource harvested sustainably, showcasing the potential for combining renewability with architectural innovation.
Importance: Utilizing renewable materials reduces the strain on non-renewable reserves, paving the way for long-term ecological balance.
Reused/Recycled
Incorporating materials from previous construction cycles can significantly extend their lifecycle. Projects like Chi-She illustrate how reclaimed bricks and timber can be creatively repurposed into functional and aesthetically pleasing designs.
Importance: Reuse and recycling lower waste output, mitigating the environmental burden of construction debris.
Reusable/Recyclable
Materials designed for easy disassembly and reprocessing are essential for creating closed-loop systems. The Recycled Glass Walls project highlights the potential of modular glass bricks that can be disassembled and reused, offering flexibility and sustainability.
Importance: Closed-loop systems minimize waste and resource extraction, aligning with circular economy principles.
Biodegradable
Biodegradable materials, such as those utilized in the Breeding Space pavilion with mycelium and straw, naturally decompose and reintegrate into ecosystems. This eliminates landfill waste while enriching the environment.
Importance: Biodegradable materials support ecosystems and reduce the ecological footprint of construction projects.
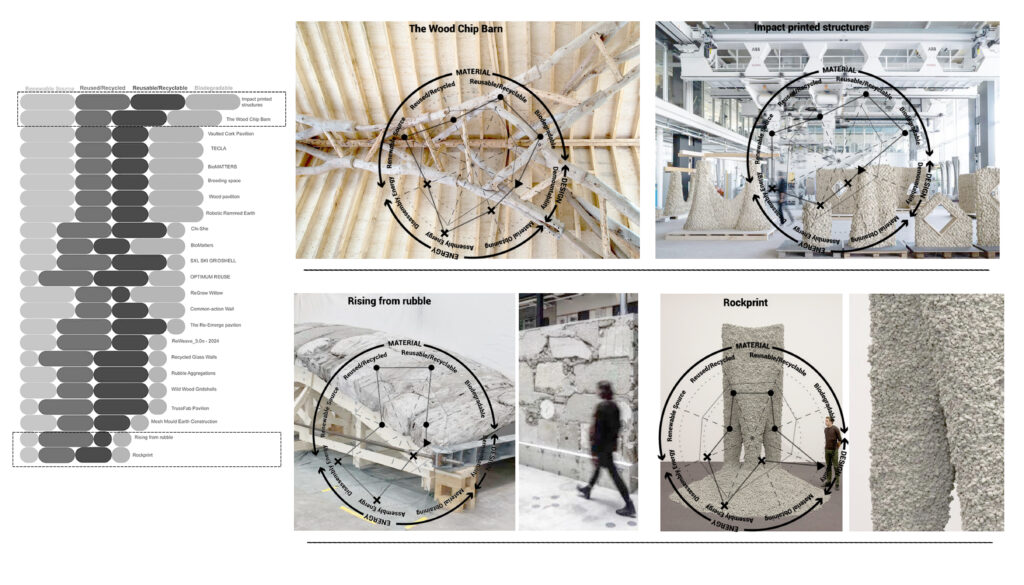
Energy Consumption
Material Obtaining
Energy efficiency in sourcing raw materials lowers the environmental cost of extraction. Projects like Mesh Mould Earth Construction leverage locally sourced earth materials, reducing transportation-related energy use.
Importance: Reducing the energy footprint of material extraction conserves resources and minimizes emissions.
Assembly
Fabrication and assembly processes can be optimized to use less energy. The ReWeave_3.0 initiative employs robotic manufacturing to upcycle textile waste efficiently, demonstrating energy-conscious assembly.
Importance: Efficient assembly processes directly contribute to the sustainability of the overall construction lifecycle.
Disassembly
Energy-efficient disassembly techniques, as seen in projects like Rubble Aggregations, ensure that materials can be reclaimed and repurposed with minimal energy expenditure.
Importance: Sustainable disassembly practices close the energy loop and enhance lifecycle efficiency.
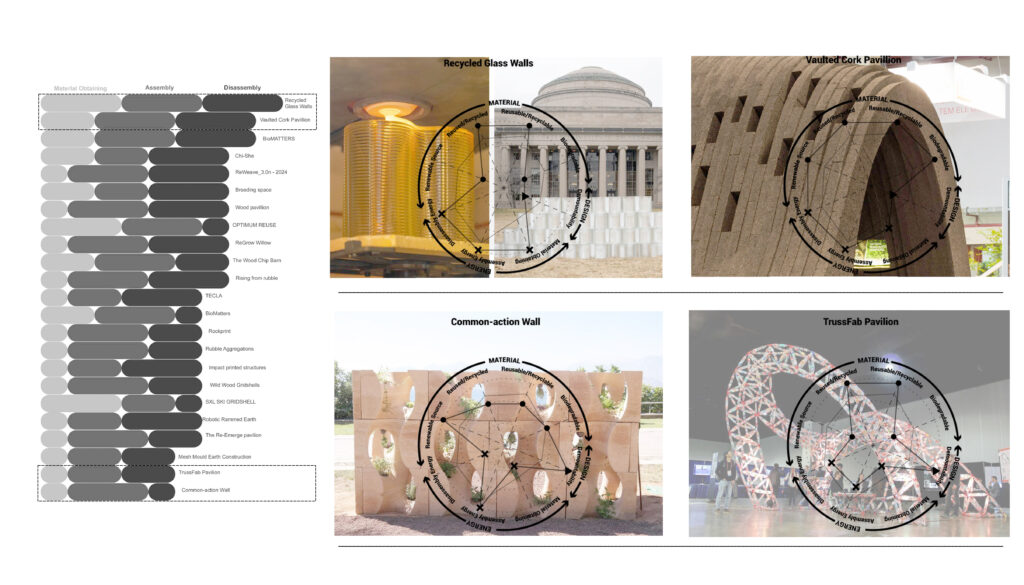
Processes and Circularity in Advanced Construction Practices
Circularity in 3D Printing (High Circularity)
3D printing techniques have shown exceptional potential for circularity by utilizing biodegradable and recyclable materials. These methods emphasize material efficiency and reduced waste.
Case Studies:
- TECLA: Utilizes natural clay, a fully biodegradable material, within a closed-loop system. Components can be rehydrated and reused, exemplifying high material circularity.
- BioMatters: Focuses on renewable and reusable biomaterials, blending ecological responsibility with innovative fabrication.
- Impact Printed Structures: Employs earth-based materials that are both biodegradable and local, ensuring a low environmental footprint.
Materials Commonly Used:
- Clay/Earth: Biodegradable, supports rematerialization.
- Mycelium: Renewable and biodegradable.
- Glass: Recyclable, though non-biodegradable.
- Concrete: Recycled aggregates with limited biodegradability.
Circularity in Pick-and-Place Techniques (Medium Circularity)
Pick-and-place methods accommodate modular materials, allowing for partial reuse and medium circularity, particularly with renewable and non-renewable components.
Case Studies:
- Chi-She: Repurposes ceramic components that are reusable and maintain aesthetic appeal.
- Vaulted Cork Pavilion: Demonstrates the use of cork, a renewable and modular material, adaptable for future designs.
- ReWeave_3.0: Innovates with textile waste, integrating recyclable fibers into modular structures.
Materials Commonly Used:
- Ceramics: Durable and reusable in modular forms.
- Cork: Renewable, recyclable, and modular.
- Textile Waste: Recyclable but complex due to mixed fibers.
- Reclaimed Timber: Recyclable and durable, offering aesthetic and structural flexibility.
Materials and Circularity Framework
High Circularity and Renewable:
- Clay/Earth: Biodegradable and rematerializable, enabling ecological integration.
- Mycelium: Combines renewability with biodegradability for innovative applications.
- Cork: Demonstrates dual attributes of renewability and recyclability.
Partially Circular:
- Recycled Glass: Fully recyclable but non-biodegradable.
- Textile Waste: Recyclable, albeit challenged by material heterogeneity.
- Reclaimed Timber: Offers longevity and reuse opportunities.
Non-Renewable and Low Circularity:
- Concrete: Incorporates recycled aggregates but has low biodegradability.
- Plastics: Non-biodegradable, with upcycling as the primary circular strategy.
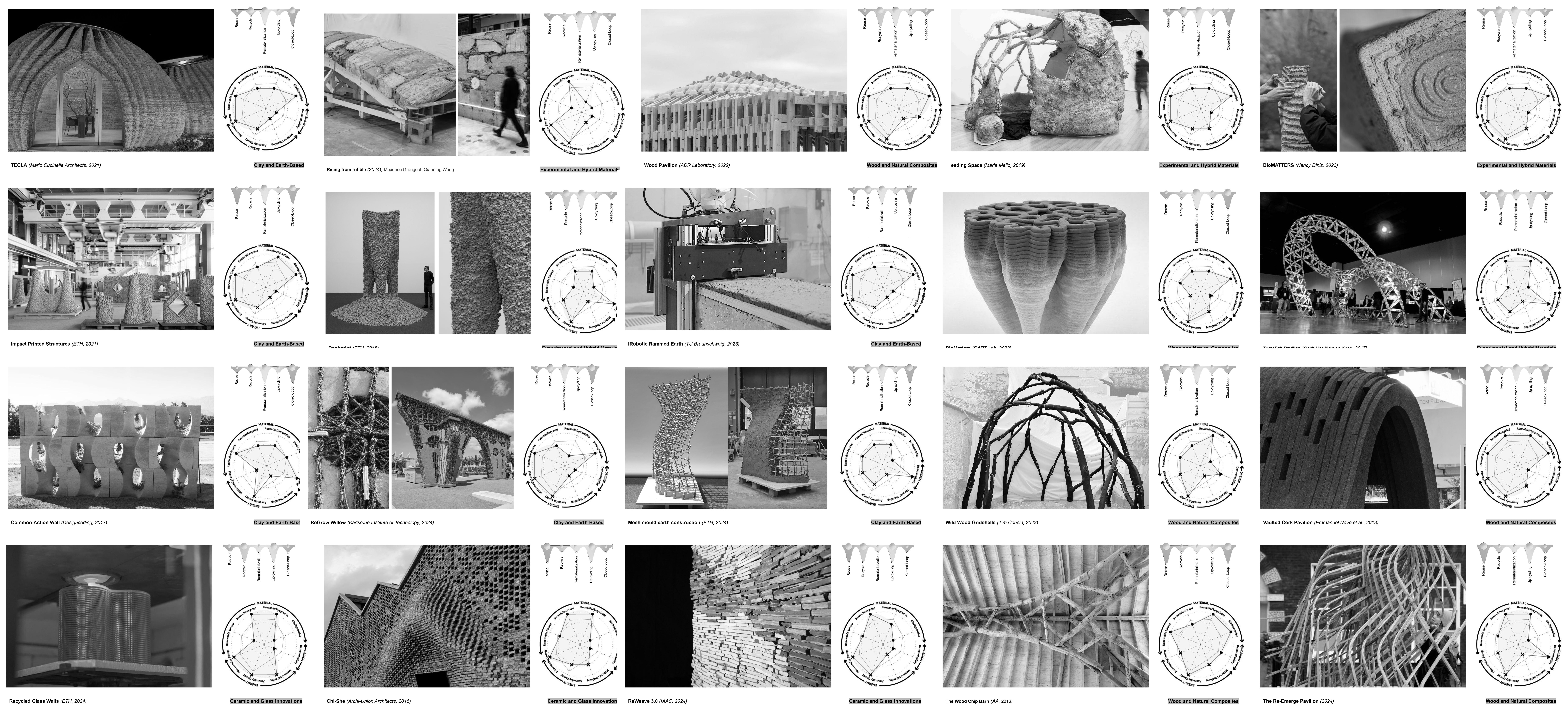
Conclusions
Integrating circularity into construction practices is a multifaceted challenge that demands both innovative fabrication methods and strategic material choices. Processes like 3D printing, with its high material efficiency and ability to use biodegradable and recyclable inputs, highlight the potential for closed-loop systems. In parallel, pick-and-place techniques offer modularity and flexibility, supporting medium circularity through partial reuse and adaptability.
Key materials such as clay, mycelium, and cork exemplify high circularity due to their renewable and biodegradable properties, while others, like reclaimed timber and recycled glass, present viable options within partially circular frameworks. Even low-circularity materials, like concrete and plastics, can play a role when integrated thoughtfully, such as through upcycling or the use of recycled aggregates.
By aligning these advanced processes with circular economy principles, the construction industry can mitigate environmental impact, promote resource efficiency, and pave the way for sustainable architectural solutions. This requires a deliberate shift towards designing systems that prioritize longevity, adaptability, and minimal waste.