Computational Structural Optimization: A Case Study of High-Rise Integration Through Parametric Design
When approaching the structural design of complex high-rise buildings, computational optimization becomes an invaluable tool in achieving both efficiency and architectural vision. In this post, I’ll walk you through a fascinating case study of how we utilized Karamba and Grasshopper to optimize a mixed-use tower’s structural system.
Project Overview
Our tower stands as a vertical city, strategically divided into three distinct programmatic zones: residential units crown the building, branded residences occupy the middle section, and a hotel anchors the lower portion. One of our primary challenges was integrating multiple sky lobbies while maintaining structural integrity throughout the tower. To tackle this complexity, we developed a systematic parametric workflow that would guide our optimization process.
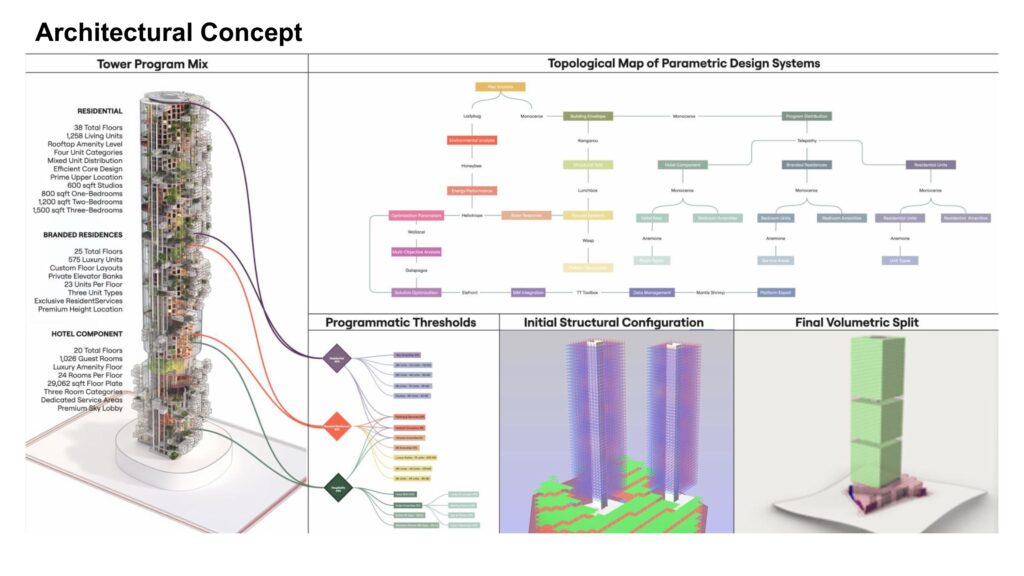
Breaking Down the Structure
The structural system consists of several key components working in harmony. At its heart, a concrete shell core provides primary stability. Around this, we developed a responsive column system that adapts to varying conditions throughout the building – from the ground-level lobby to the double-height sky lobbies and standard floors. The facade serves dual purposes: architectural expression and wind load management, while mesh-based floor plates distribute loads at each level.
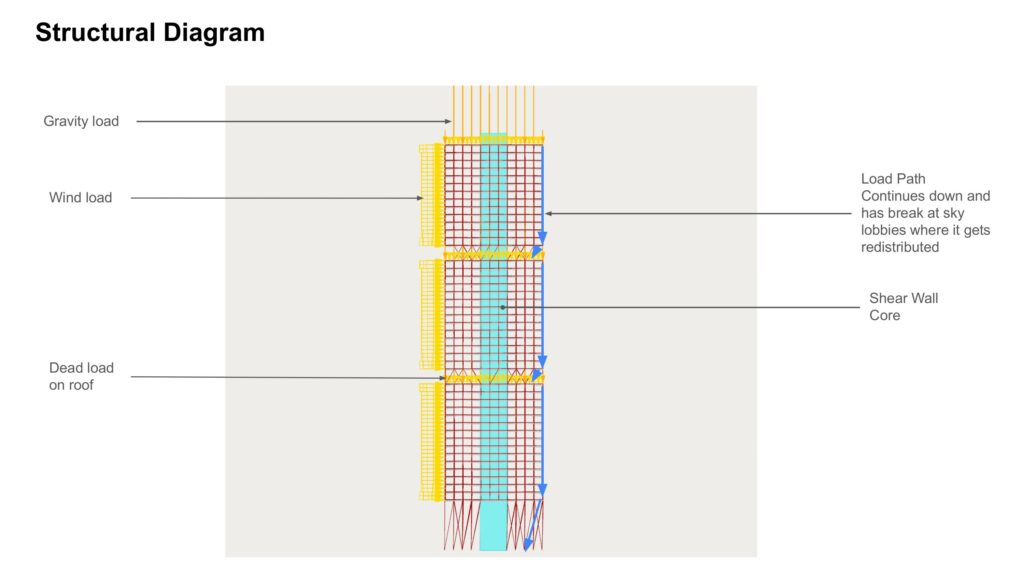
Load Path Analysis
Understanding load paths was crucial to our optimization strategy. The structure manages three primary force types:
- Wind loads acting on the facade
- Gravity loads traveling through the structural elements
- Dead loads from the roof system
The sky lobbies presented interesting challenges as major load transfer points, with the shear wall core playing a crucial role in maintaining stability.
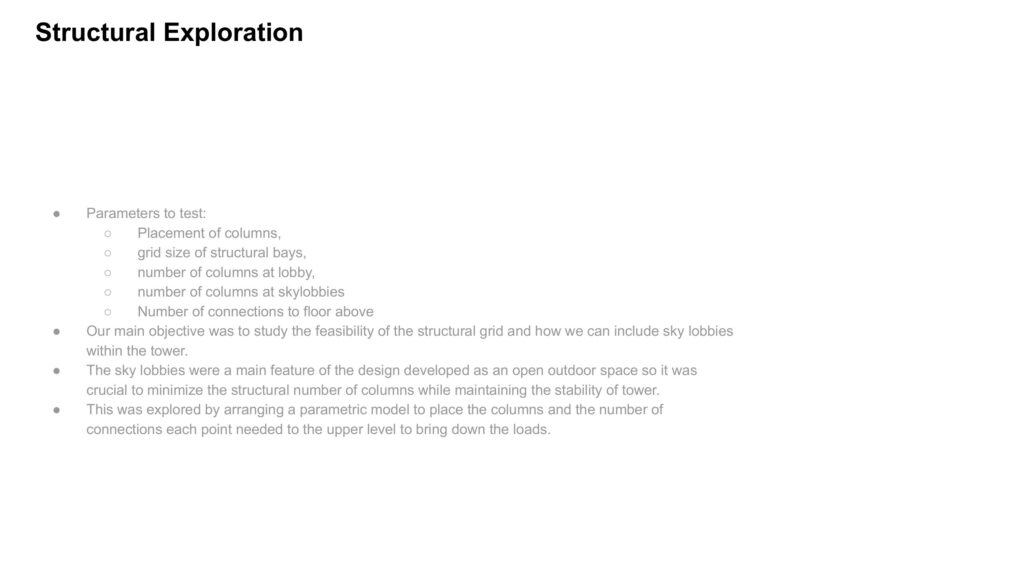
Three-Step Optimization Process
Our optimization journey followed a methodical three-step approach that progressively refined our solution:
Step 1: Single Objective Optimization
We began with a straightforward displacement minimization strategy. Our initial results showed a displacement of 406cm – a starting point that demanded improvement.
Step 2: Cross-Section Refinement
By implementing cross-section optimization and logical element grouping, we significantly improved performance, reducing displacement to 124cm.
Step 3: Multi-Objective Optimization
The final step employed a sophisticated multi-objective approach considering displacement, element count, and carbon footprint simultaneously. This resulted in a balanced solution with approximately 32,850 elements and a carbon footprint of 19.7 million kgCO2e.
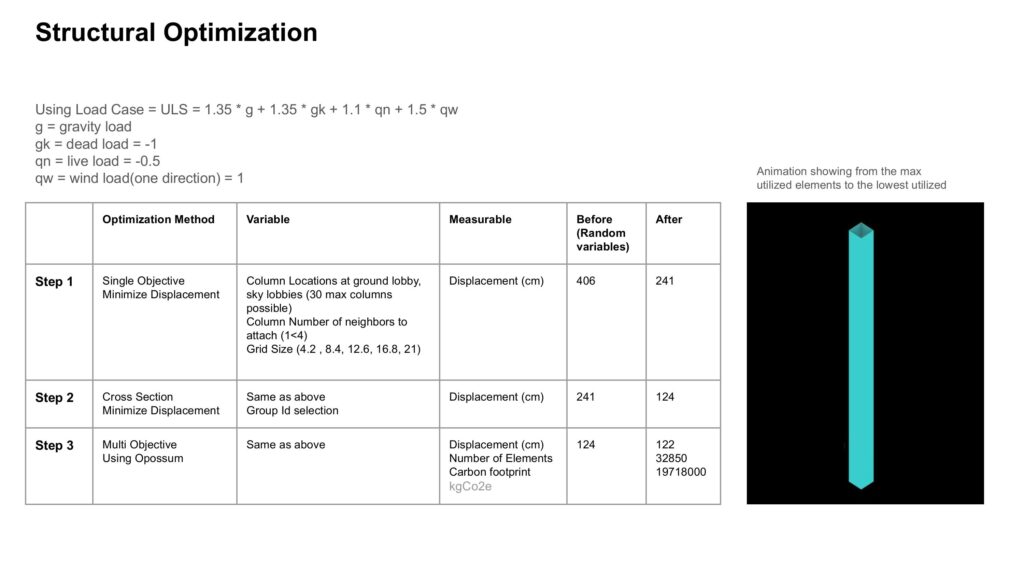
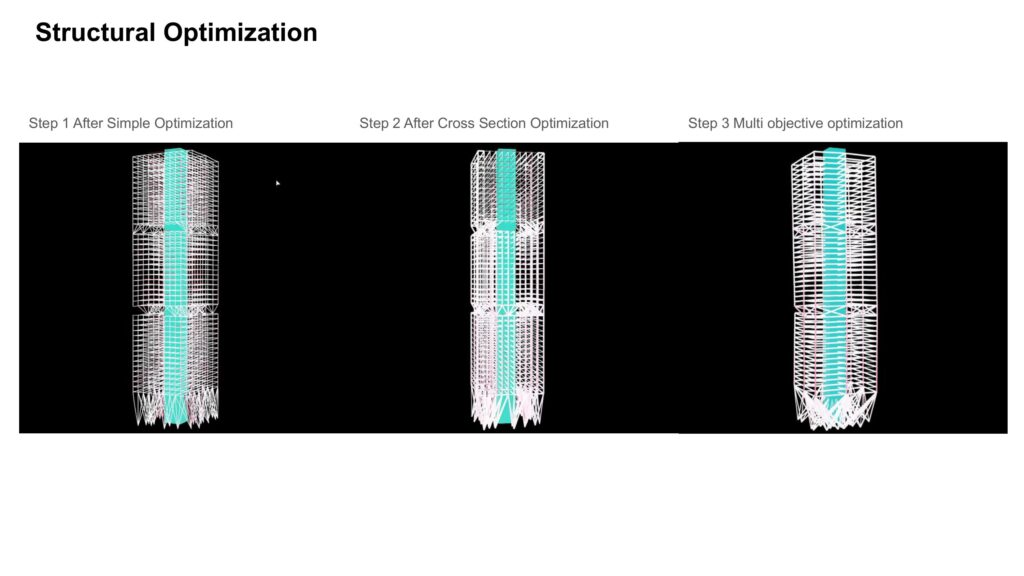
Grid Configuration Studies
Our grid studies revealed interesting trade-offs between efficiency and flexibility. After extensive analysis, we settled on a 4.2m x 4.2m grid configuration. While this increased our element count fourfold compared to larger grid options, it only resulted in a 12% increase in carbon footprint. This decision provided superior floor layout flexibility and better control over structural elements.
[Image Break – Grid study comparison diagrams]
Methodology and Evolution
The optimization process followed a clear progression:
- Initial column placement and basic structural layout
- Cross-section optimization for improved performance
- Multi-objective optimization using Opossum to balance multiple parameters
[Image Break – Form evolution diagram showing methodology progression]
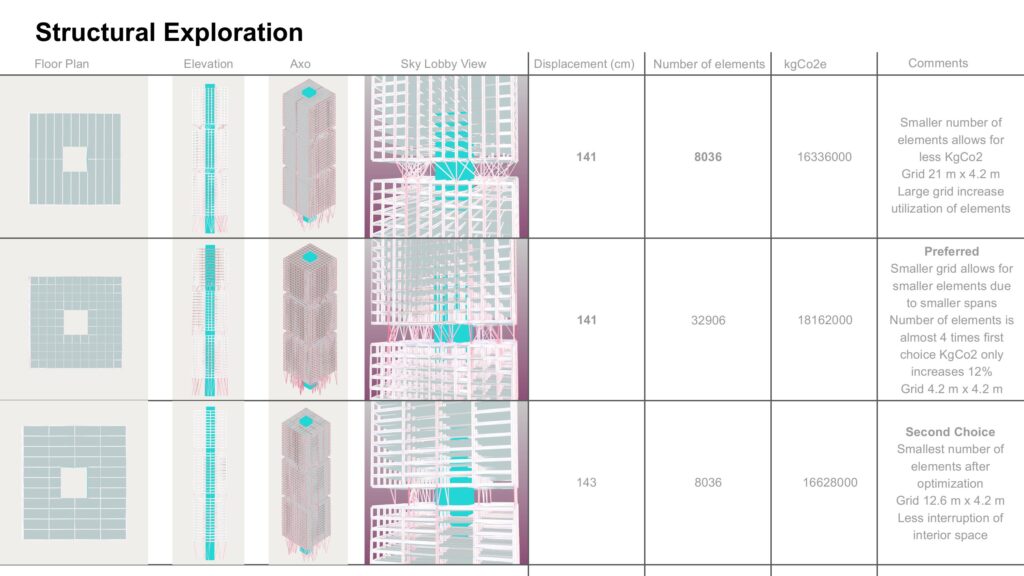
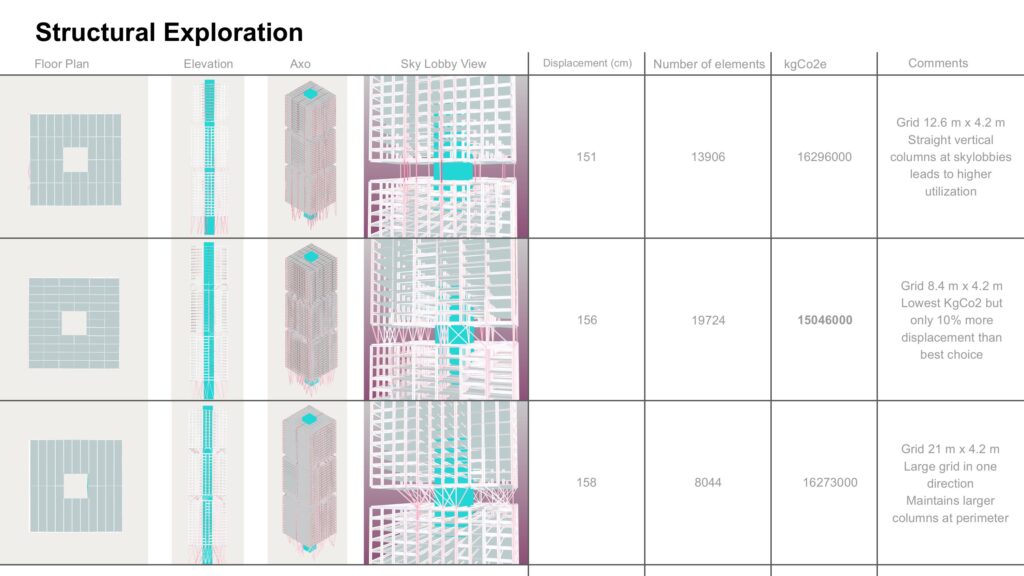
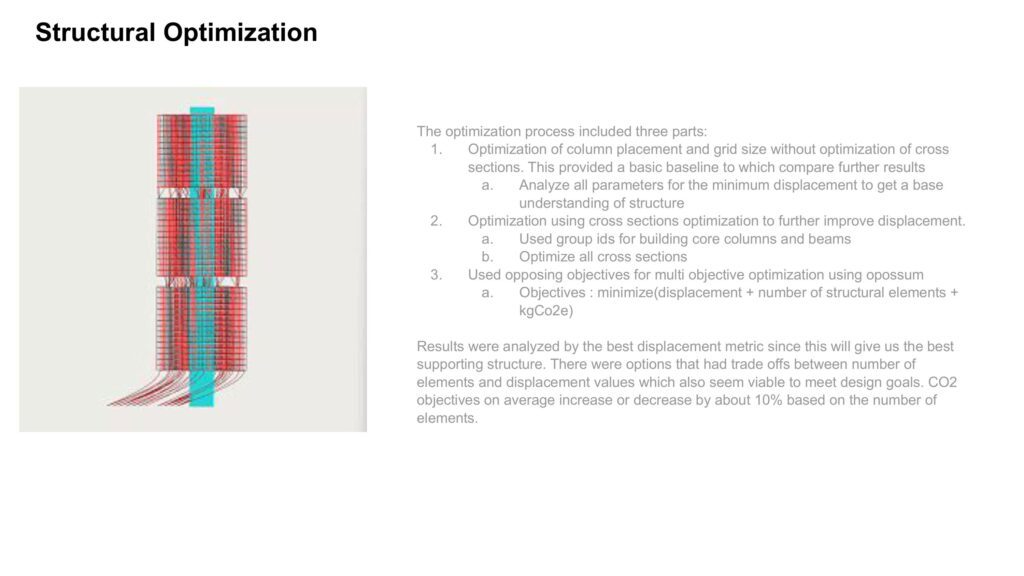
Conclusions and Future Developments
Our multi-objective optimization approach successfully identified optimal solutions for grid sizing that balanced element count and carbon footprint. However, several challenges remain under investigation:
- High-utilization elements requiring further optimization
- Sky lobby stability enhancement
- Load transfer refinement through sky lobbies
- Beam stability improvements
- Column size optimization through enhanced bracing strategies
This case study demonstrates how computational structural optimization can help navigate complex architectural and engineering challenges while maintaining focus on sustainability and efficiency goals.
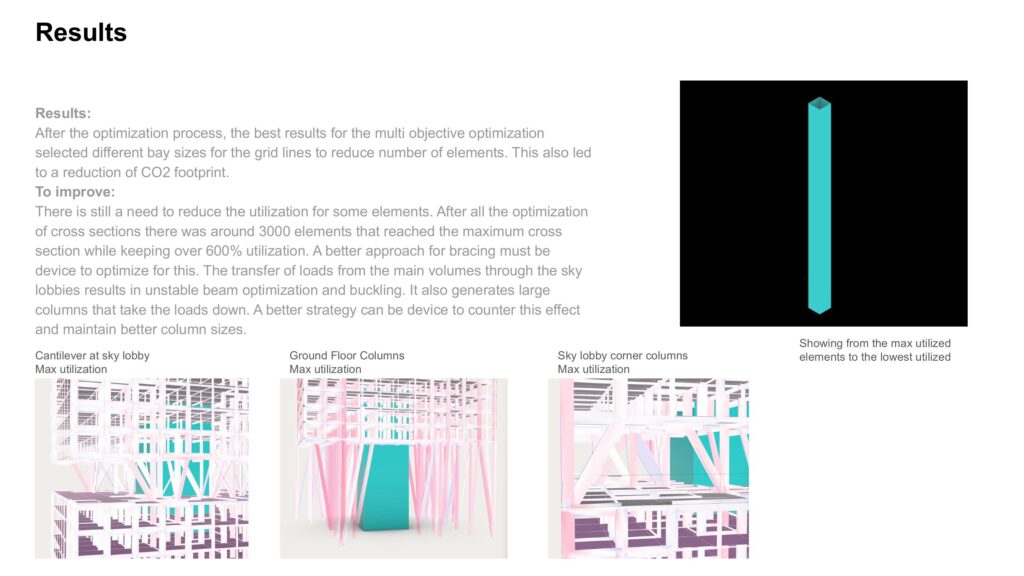