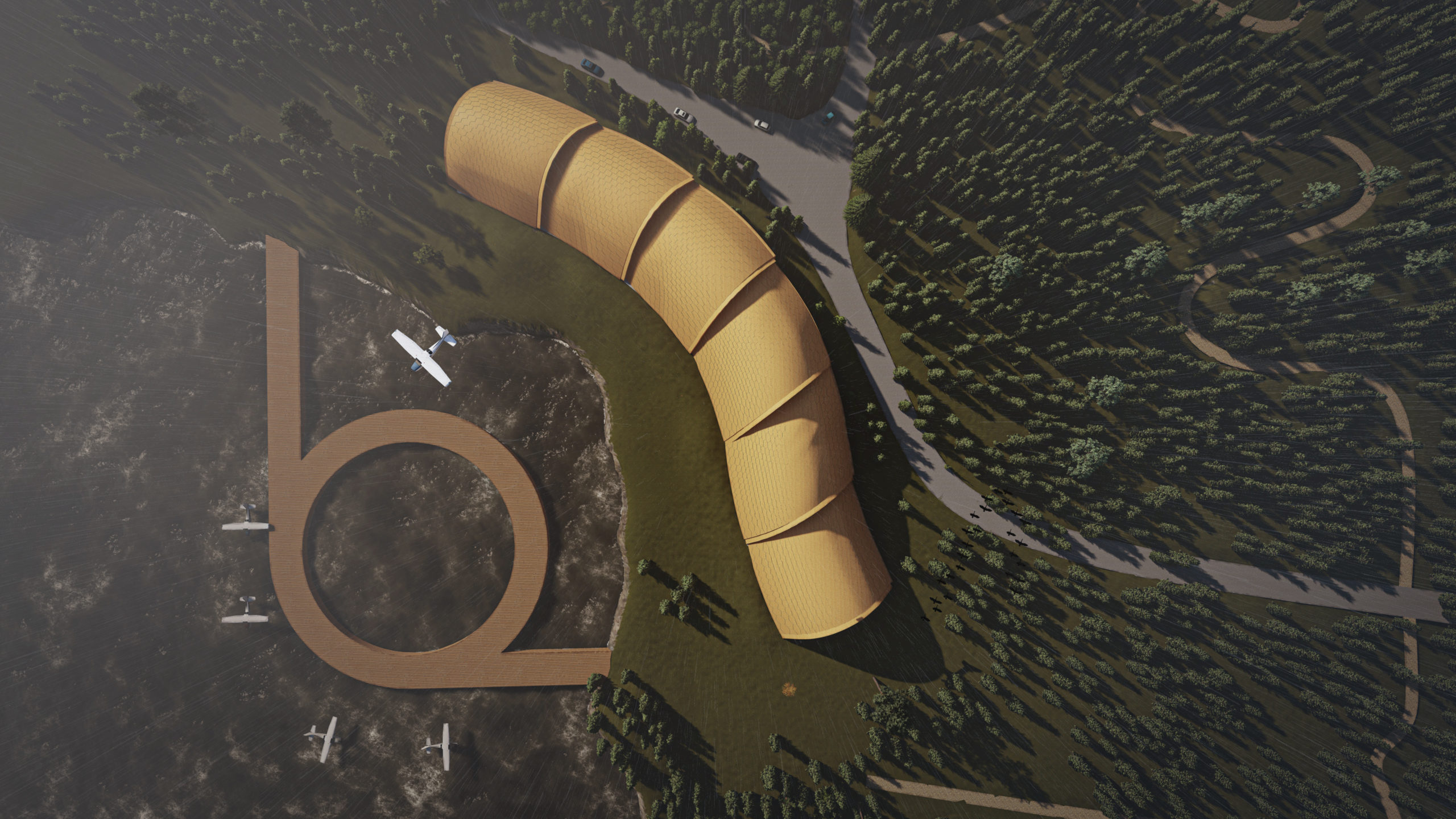

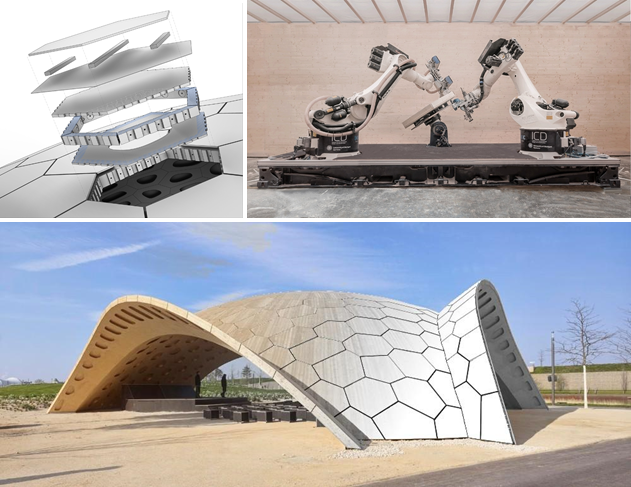
Structural System Description
Segmented timber shells take advantage of the structural performance of double curved surfaces formed from hex-dominant panels. Traditionally these have been formed from concrete, but recent advances in engineered timber materials, parametric design and precision robotic fabrication have seen the development of this structural system in the last 10 years. Examples include the Landesgartenschau Exhibition Hall2, HexBox Canopy3, Recycleshell4, the livMatS Building5 and the BUGA Wood Pavilion6.
Comparing the performance of the first architectural scale example of the Landesgartenschau Exhibition Hall which achieved a span of 17 x 11 m using solid panels, the hollow cassettes of the BUGA Wood Pavillion achieved a span of 30 m using the same volume of material per square meter.
Another major advantage of the hexagonal panel system is that the load is distributed between elements, simplifying connection design significantly when compared with triangular or quad grids that require specialized joints; in fact the HexBox Canopy project utilized a mortise and tenon joint and was assembled by university students on campus over the course of two weeks.

System Planarization
One of the critical problems with segmented timber shells, is to provide planar panels so that the structure can be fabricated from flat stock. Here we show some early experiments with planar solutions for anticlastic (top) and mixed surfaces (bottom).


The Challenge of Planarization
Designing hexagonal segmented timber shell structures poses a significant challenge in the requirement that the panels are planar, which allows fabrication from flat stock. This results in constraints on the structural system, in particular the limits of curvature, segmentation size, and mixtures of Gaussian curvature which can cause the segmentation system to break down. The key to achieving planarity is the generation of the intial hex mesh. Recent work provides a framework for the initial mesh which is generally planarizeable7, wherein a tri-mesh is aligned with the principal curvature directions of the underlying surface, and the hex mesh taken from the dual.
Two planarization algorithms were explored for their ability to generate planar hex meshes:
Although the ABxM method provides finer control and the ability to individually adjust vertices, the nGon method was found to be more reliable. Designers must also understand that the process of planarizing the hex mesh results in some deviation from the starting surface. This can be somewhat overcome by the orientation of the mesh edges and anchor points provided. Additional constraints that must be considered at the design stage are the dimensions of source material and the range of robot articulation.

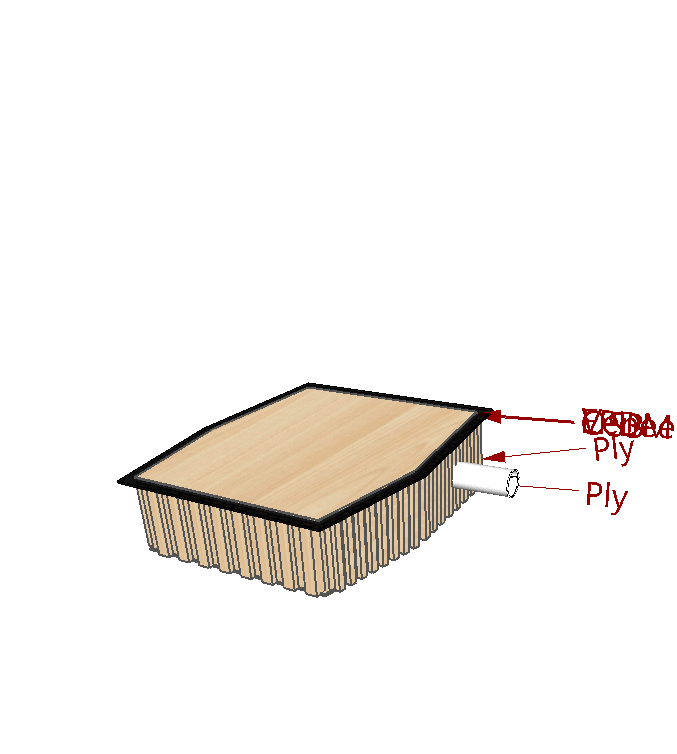
System Materialization
We follow the BUGA Wood Pavillion and use hollow cassettes formed from engineered timber flat stock. Precision machined finger jointed sides connect the top and bottom sheets, with plywood used for the internal plate, and OSB used for the concealed plate. The void of the box provides space to run services and insulation. Over this an EPDM waterproofing membrane is installed, with a veneer fascia panel on top. We have not shown the spacers between the EPDM and the veneer requied for draining. Stainless steel wood screws hold the panel assembly together and join neighbouring panels.
Unit costs for these materials were assessed as follows:
- 6 mm Veneer: 28 CAD/m2
- 1.9 mm EPDM: 80 CAD/m2
- 18.5 mm OSB: 36 CAD/m2
- 18.5 mm Ply: 61 CAD/m2
- 36 mm #10 wood screw: 0.17 CAD/ea.
Additionally it is estimated that two six-axis robots are required for a period of 6 months, with an estimated cost to hire of 700k CAD.

Project Site
Our project is located in Tahsis, BC a former timber milling town that has become dilapidated since the closure of the sawmill in 2001, with a population decline of 90%. We aim to reinvigorate the town by providing a float plane terminal for access for tourism, attracted by the wildlife that has returned to the inlet since pollution from the mill ceased. Humpback whales in particular are a draw to the area. The project site is located on the waters edge and intends to provide flood protection for the town.
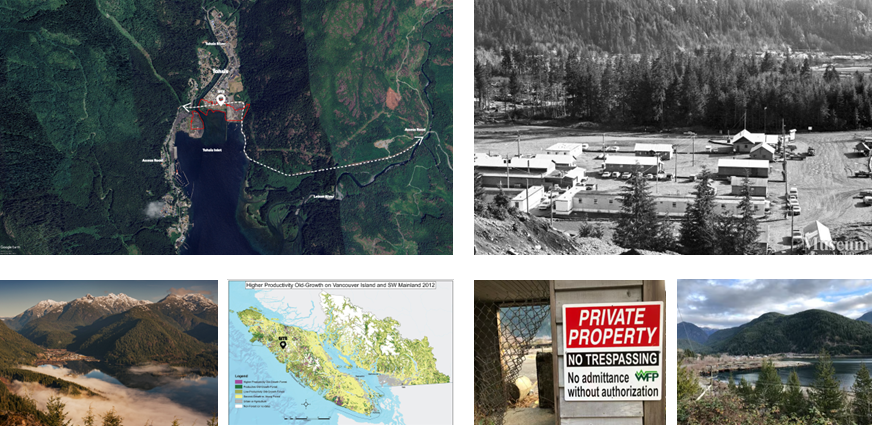
Site Analysis
The Tahsis flood risk assessment report, provided us with a comprehensive understanding for new floodplain mapping and also the planning for floods that might happen in the near future. The study is based on a two-dimensional hydrodynamic computer model with a LiDAR digital elevation model.
We initiated an exploration of methods to simulate and analyze water flow across our site’s surface. Leveraging MapWinGIS, we obtained topographical data and synchronized it with Open Street Maps using our site’s coordinates. Then we started looking into examining various flow patterns and potential locations for our intervention.
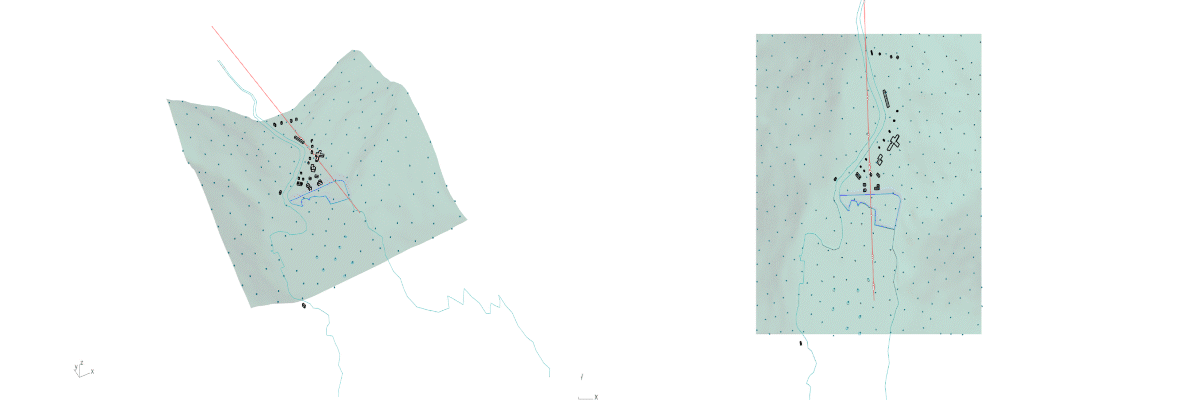
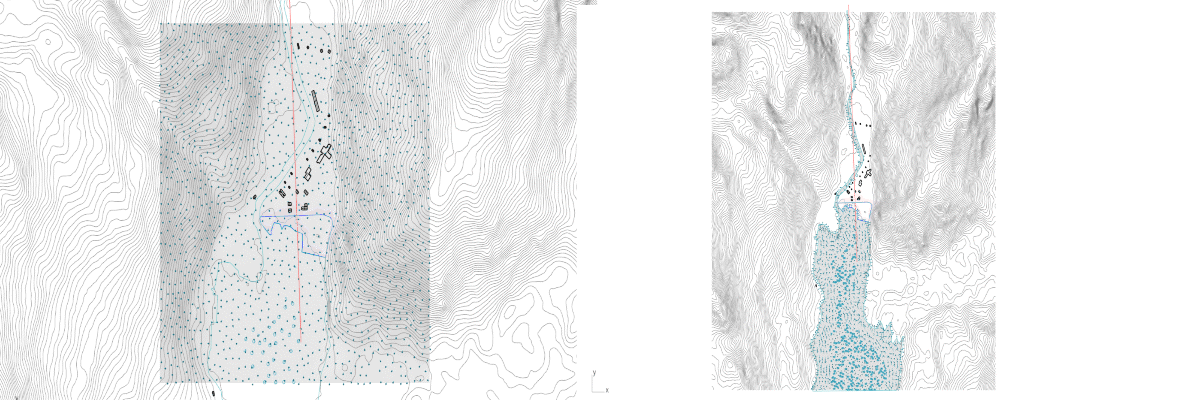
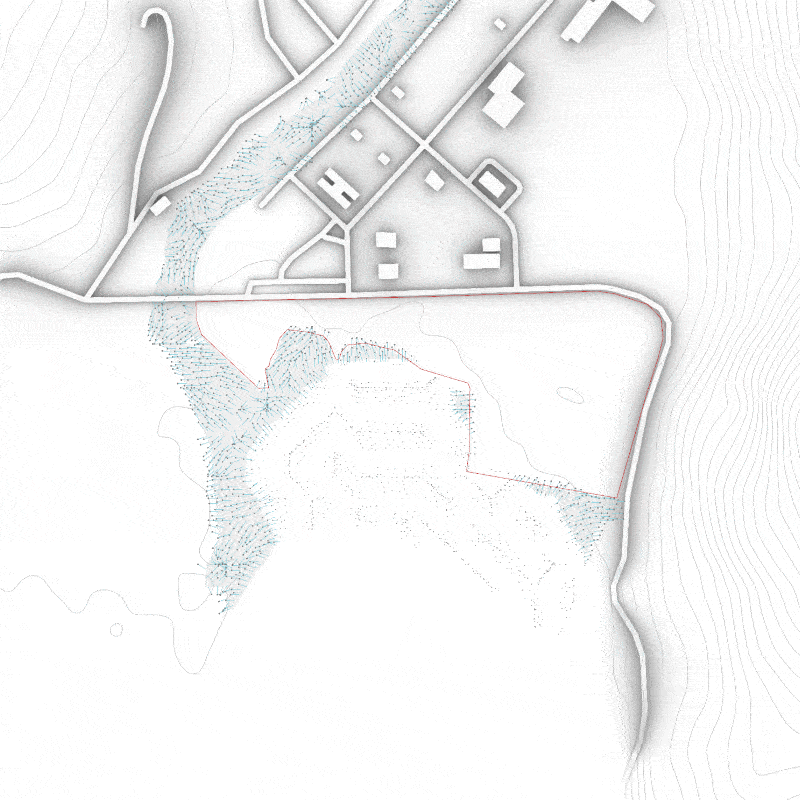
Above, the diagram illustrates the water flow on our site through the deployment of 3500 vectors. This enabled us to identify and cluster high-risk areas, guiding us to the optimal placement of our building to proactively mitigate the risk of future floods.
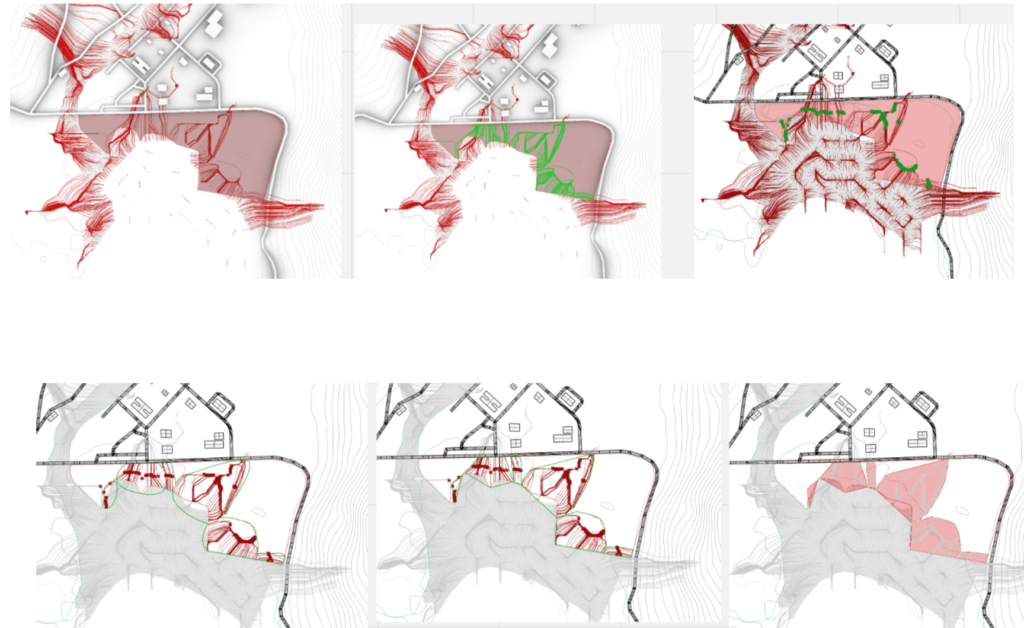

Based on the need to provide access to the water for the program of a float plane terminal and a desire to provide an artificial berm the decision was taken to locate the building toward the waters edge. Flood modelling indicates that this arrangement protects the area behind the building which can then be used to pick up and drop off areas as well as landscaping. This orientation was also selected for it’s advantageous southern aspect that can provide natural day lighting via a clerestory arrangement.
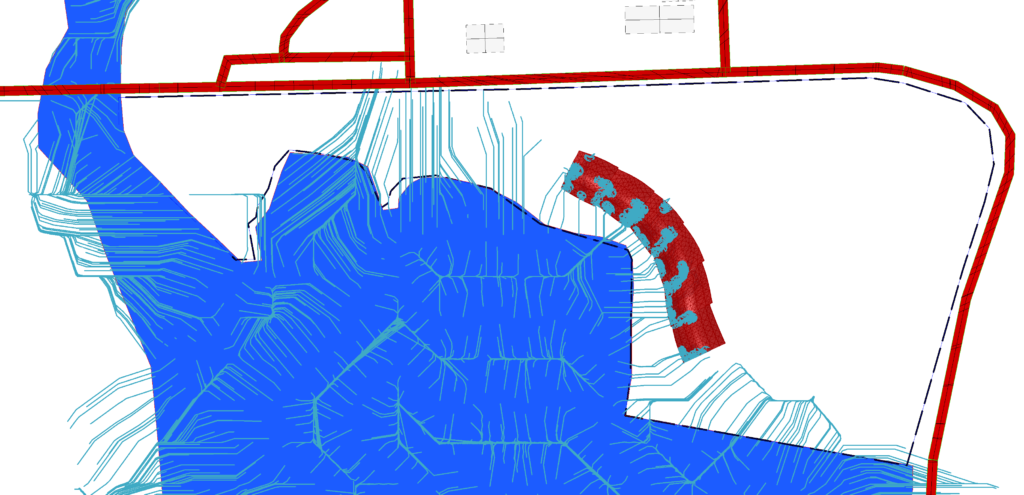
Program
Due to the limited access into the town, we decided to design a float terminal on the shoreline that can help increase the tourism and access potential of the town, whilst also protecting the town from high tides flooding from the shoreline. Therefore, the structure is closed on the south side facing the water and open on the north facing the community with clerestories along the East-West axis for solar penetration.
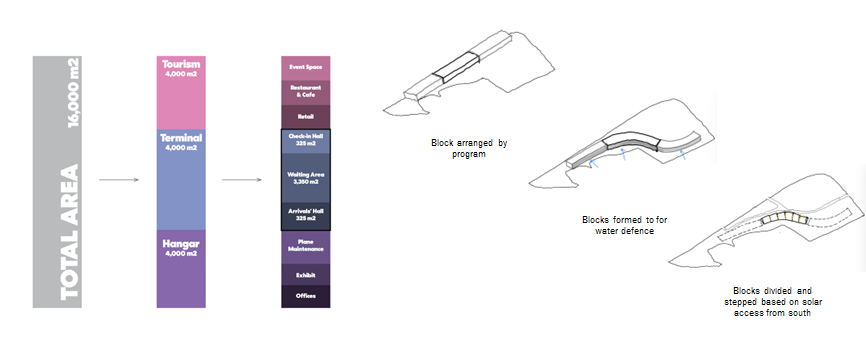
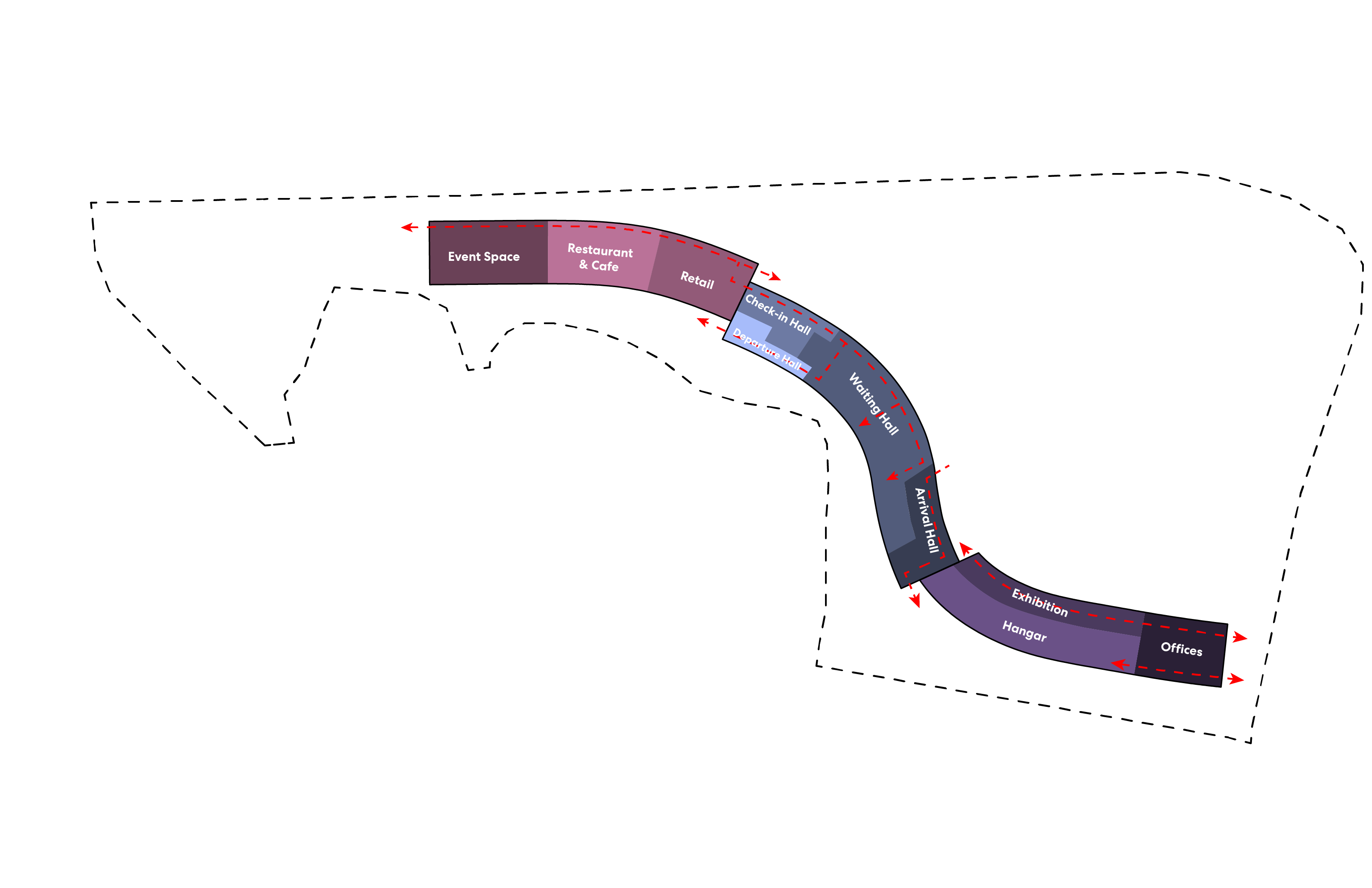
Material Traceback
Examining the material resource use for the structural system we find that the source materials are timber, steel and petrochemicals. Suppliers were located in North America for the flatstock, adhesive and membrane. Cost information was gathered from RS Mean and embodied carbon data taken from supplier or industry Environmental Product Declarations (EPDs)14,15,16. Material resource use is summarised as follows:
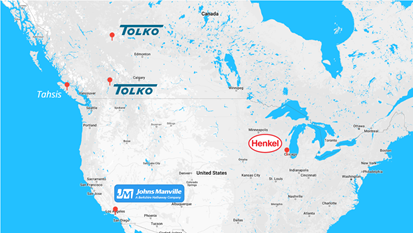
- Ply / veneer:
- Quantity: 3,922 m2
- Cost: $668,900
- Delivery distance: 825 km
- Transport emissions: 6,370 kg CO2e
- A1 – A3 emissions: 78,800 kg CO2e
- OSB
- Quantity: 1,485 m2
- Cost: $53,500
- Delivery distance: 1,591 km
- Transport emissions: 1,760 kg CO2e
- A1 – A3 emissions: 6,600 kg CO2e
- EPDM
- Quantity: 1,485 m2
- Cost: $118,800
- Delivery distance: 2,419 km
- Transport emissions: 517 kg CO2e
- A1 – A3 emissions: 13,160 kg CO2e
- Totals
- Cost: $841,200
- Embodied Carbon: 107,207 kg CO2e
For the EPDM we also examined sourcing from Jinan, Shandong, China, and although the transportation emissions were reduced (0.19 kg CO2e/m2 compared to 0.35 kgCO2e/m2) , the A1 – A3 emissions were found to be much higher (26.3 kg CO2e/m2 compared to 8.9 kg CO2e/m2).
For our project we deliberately exclude any benefit from biogenic carbon sequestration, firstly because the self regulated Canadian forestry industry continues to log old growth forests and practices clearfelling, and secondly, due to the questionable assumptions that underpin the ISO 14040 rules regarding biogenic carbon accounting, that are not borne out by the research, in particular, we draw the reader’s attention to recent research from the World Resources Institute on this topic along with this Nature paper from the same authors.
Architecture Drawings
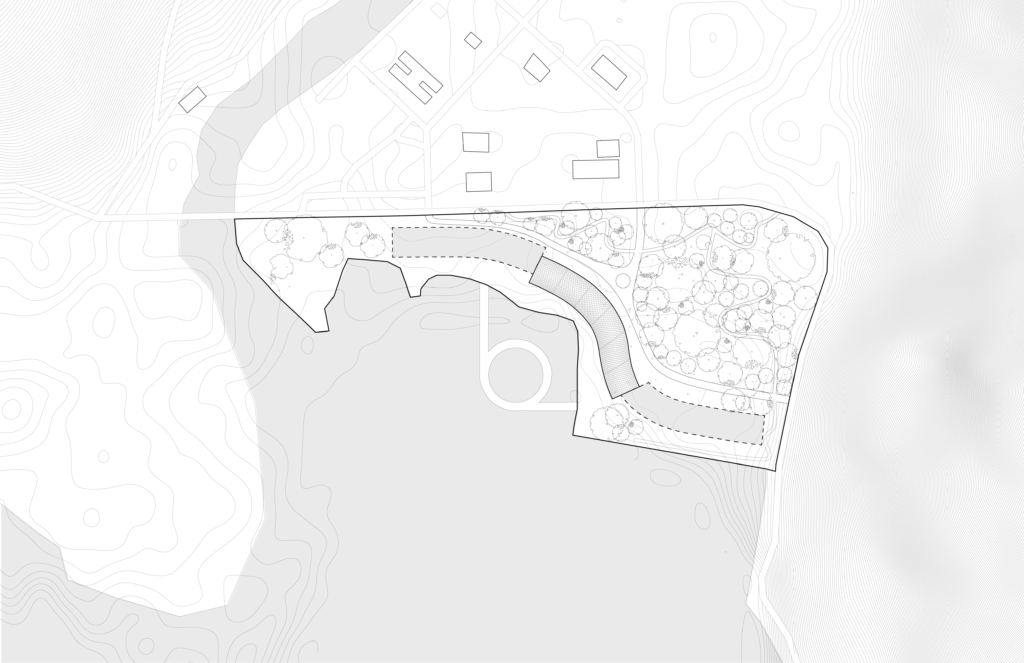
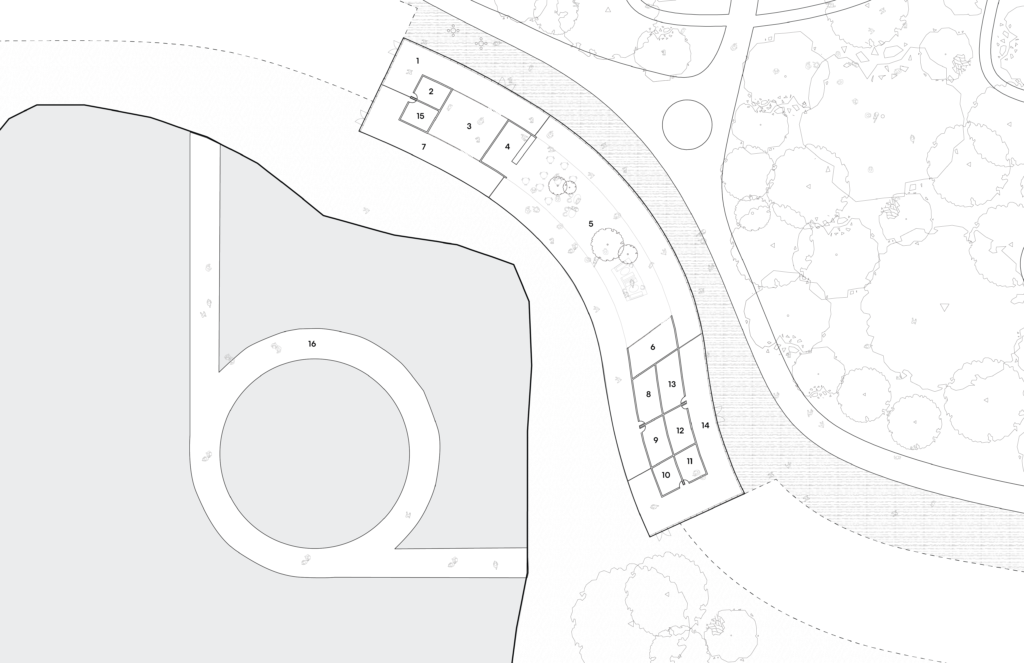
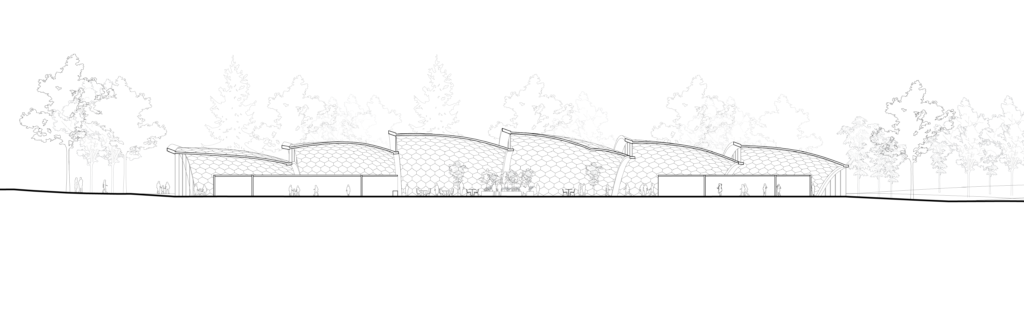
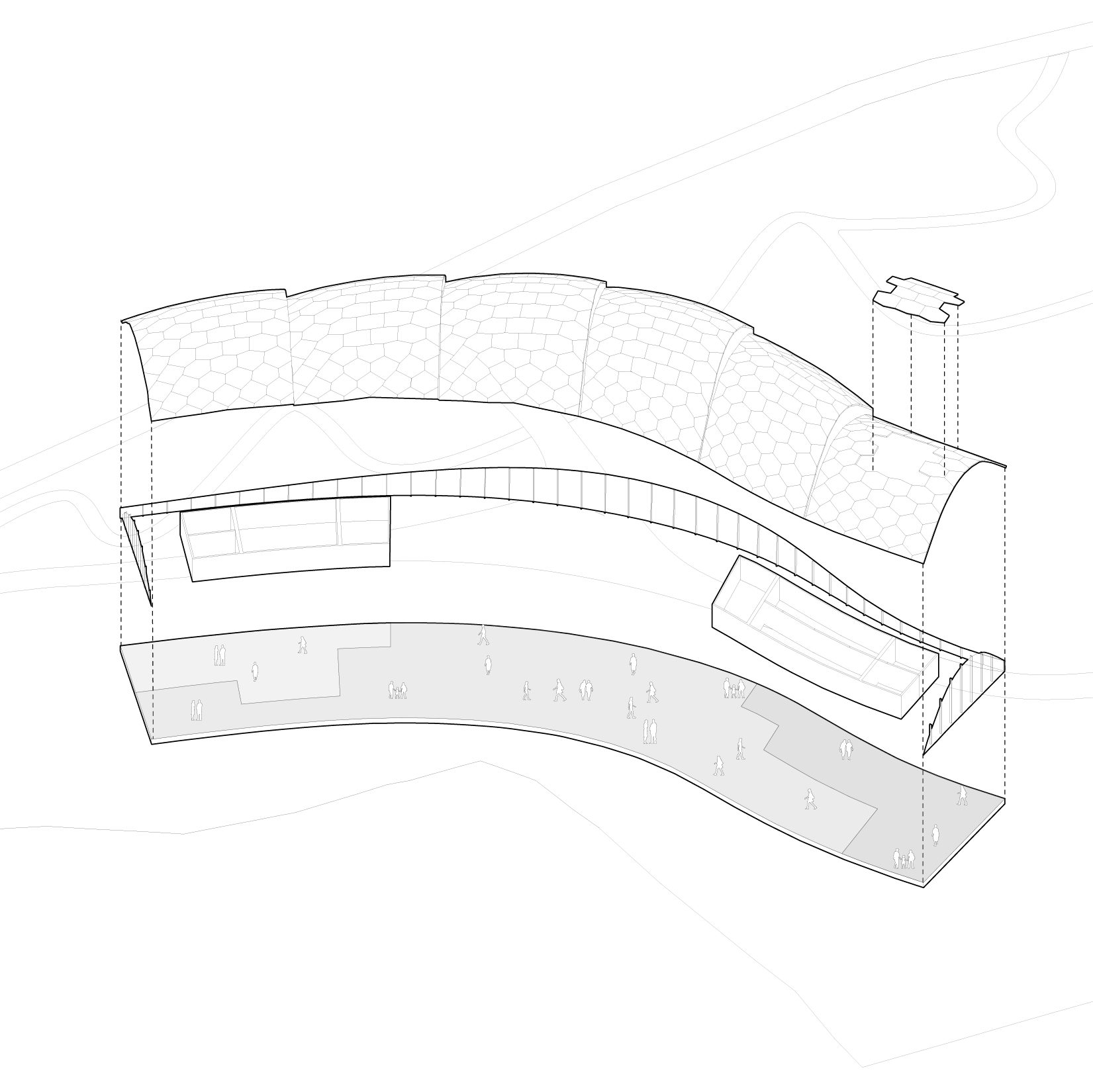
Final Renders
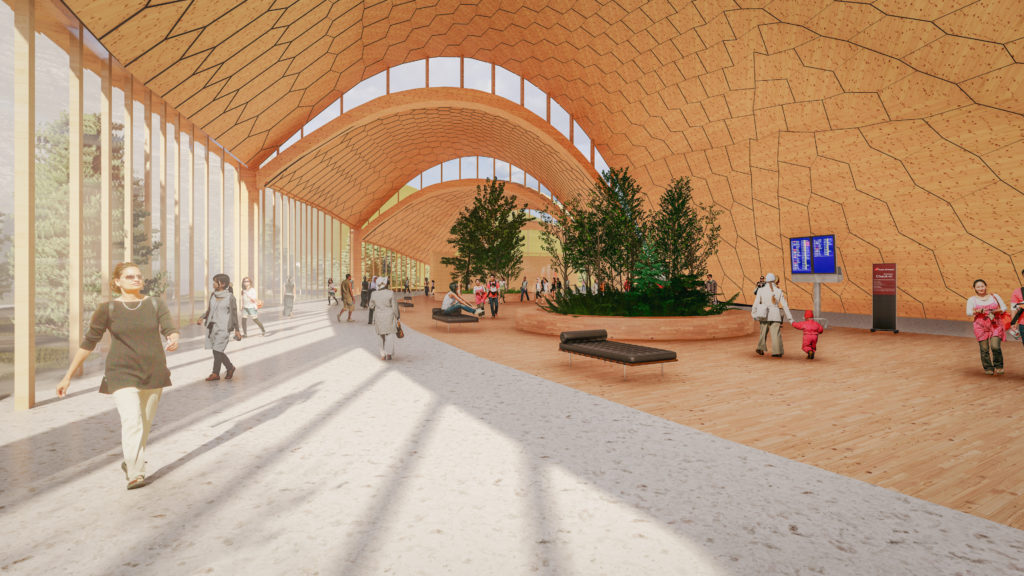
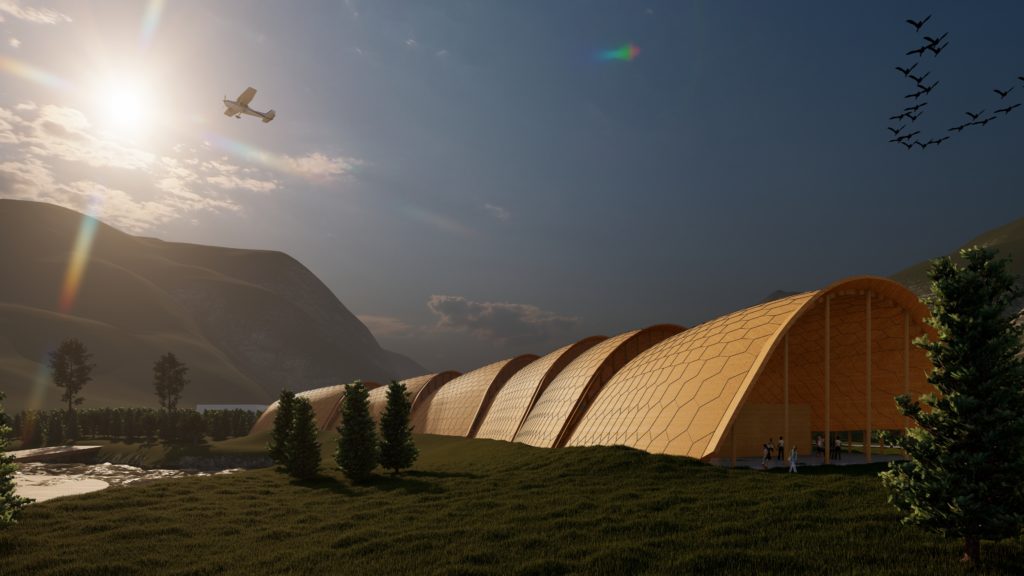
A comment regarding timber sustainability
Although timber is often better than current steel and concrete options, the picture is enormously complex. Timber can be sustainable, but it requires care from the project team to ensure that it is sourced from sustainably managed plantations – forestry certification schemes can help, but are self regulated and are insufficient to guarantee that your timber is sourced responsibly. Timber supply chains are notoriously corrupt, with old growth timber being labelled FSC. Impacts to the environment are documented in Environmental Produce Declarations (EPDs) and include global warming (embodied carbon), acidification, eutrophication, ozone depletion and material resource consumption. However, the rules around EPDs (so called Product Category Rules) are written by industry and can be gamed to produce certain outcomes. Evidently the answer to the question Does timber sequester carbon? depends very heavily on who you ask: a forestry researcher or a climate researcher. Forestry researchers tend to look at trees in terms of linear feet of boards and use those metrics to evaluate carbon in the forest, whereas climate researchers measure the flux of carbon in the forest, which accounts for soil carbon sequestration17. Forestry researchers assert that forests reach a maximum carbon density of ~100 T/ha, after which carbon sequestration tapers off to a steady state after 40 – 80 years, however climate researchers have measured that forests increase in carbon sequestration as they age up to and beyond 400 years18,19. Trees carbon sequestration rates accelerate as their leaf canopy increases, they are continuously pumping carbon below grown in the form of carbohydrates that are exchanges with the microrhizal network for nutrients. Forestry researchers also assert that young forests are less prone to fire than old forests, however climate researchers find the opposite, and in fact forestry practices greatly increase the likelihood of forest fires, introducing and spreading pests and disease, monoculture clonal replanting, clearing for roads and providing access to humans who cause the majority of forest fires20. Another consideration is the percentage of timber fiber that makes its way into long-lived building products, with estimates ranging from 20 to 70%, this has an important bearing on the difference between the biogenic sequestration value provided on an individual product EPD, and the net change in forest biogenic carbon, but needs to be considered when selecting sustainable timber21,22. When considering the time value of the carbon released due to timber harvest the impact is of the same magnitude as emissions from agricultural expansion23,24.
By utilizing waste timber material from other production processes, composite timbers increase the percentage of timber making its way into long lived products and hence improve the overall sequestration (so long as the original timber was sourced sustainably).
Other options for fiber sources of composite materials include agricultural wastes and bamboos, combining these short rotation crops with long lived products in the built environment are estimated to increase sequestration of CO2 over timber products by upwards of 200%25. Yet another option worth considering is recycled cotton fiber, with the potential to divert waste from the third world, that releases tonnes of methane to the atmosphere as it decomposes. We considered these options early in the project, however were unable to meet the brief due to a lack of suppliers.
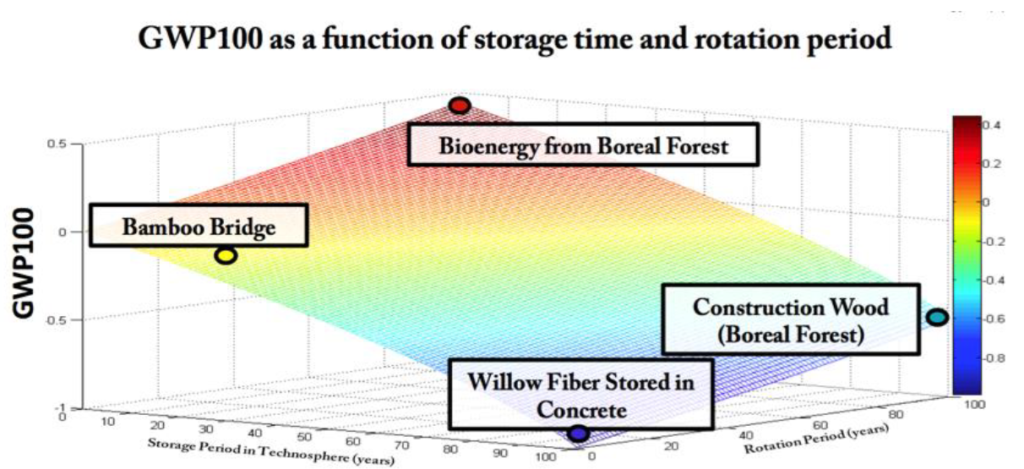
- Images from https://www.icd.uni-stuttgart.de/projects/buga-wood-pavilion-2019 ↩︎
- Menges, A., Schwinn, T., & Krieg, O. D. (2015). Landesgartenschau Exhibition Hall. Interlocking Digital and Material Cultures, 55-71. ↩︎
- Robeller, C., Barata, E. D. O., Tagliaboschi, E. V., & Schmidt-Kleespies, F. (2022). HexBox Canopy: a segmented timber plate shell with hardwood wedge joints. Journal of the International Association for Shell and Spatial Structures, 63(4), 221-231. ↩︎
- Robeller, C., & Von Haaren, N. (2020). Recycleshell: wood-only shell structures made from cross-laminated timber (CLT) production waste. Journal of the International Association for Shell and Spatial Structures, 61(2), 125-139. ↩︎
- Lauer, A. P. R., Benner, E., Stark, T., Klassen, S., Abolhasani, S., Schroth, L., … & Sawodny, O. (2023). Automated on-site assembly of timber buildings on the example of a biomimetic shell. Automation in Construction, 156, 105118. ↩︎
- Bechert, S., Sonntag, D., Aldinger, L., & Knippers, J. (2021, December). Integrative structural design and engineering methods for segmented timber shells-BUGA Wood Pavilion. In Structures (Vol. 34, pp. 4814-4833). Elsevier. ↩︎
- Pluta, K., Edelstein, M., Vaxman, A., & Ben-Chen, M. (2021). PH-CPF: Planar hexagonal meshing using coordinate power fields. ACM Transactions on Graphics (TOG), 40(4), 1-19. ↩︎
- Schwinn, Tobias; Groenewolt, Abel; Nguyen, Long; Siriwardena, Lasath; Alvarez, Martín; Reiner, Alexander; Zorn, Max Benjamin; Menges, Achim, 2023, “ABxM.PlateStructures: Agent-based Architectural Design of Plate Structures”, https://doi.org/10.18419/darus-3438, DaRUS, V3 ↩︎
- Schwinn, T., Siriwardena, L., & Menges, A. (2023). Integrative Agent-Based Architectural Design Modelling for Segmented Timber Shells. Also of interest, 177. ↩︎
- Petras Vestartas, & Aryan Rezaei Rad. (2021). NGon (v2.4.5). Zenodo. https://doi.org/10.5281/zenodo.4550592 ↩︎
- https://gallery.crmuseum.ca/photograph/tahsis-logging-camp-zeballos ↩︎
- https://thenarwhal.ca/at-the-end-of-the-forest-a-former-vancouver-island-mill-towns-struggle-for-reinvention/ ↩︎
- https://ancientforestalliance.org/ancient-forests/before-after-old-growth-maps/ ↩︎
- https://cwc.ca/en/why-build-with-wood/sustainable/green/epds/ accessed 2023-12-28 ↩︎
- https://pcr-epd.s3.us-east-2.amazonaws.com/805.SPRI_EPDM_Reinforced_EPD.pdf accessed 2023-12-28 ↩︎
- https://www.ecta.com/wp-content/uploads/2021/03/ECTA-CEFIC-GUIDELINE-FOR-MEASURING-AND-MANAGING-CO2-ISSUE-1.pdf accessed 2023-12-28 ↩︎
- Campioli, M., Malhi, Y., Vicca, S. et al. (2016) Evaluating the convergence between eddy-covariance and biometric methods for assessing carbon budgets of forests. Nat Commun 7, 13717. https://doi.org/10.1038/ncomms13717 ↩︎
- Forrester, D.I. Does individual-tree biomass growth increase continuously with tree size? Forest Ecology and Management
Volume 481, 1 February 2021, 118717 https://doi.org/10.1016/j.foreco.2020.118717 ↩︎ - Stephenson, N., Das, A., Condit, R. et al. Rate of tree carbon accumulation increases continuously with tree size. Nature 507, 90–93 (2014). https://doi.org/10.1038/nature12914 ↩︎
- Lindenmayer, D.B., Hunter, M.L., Burton, P.J. and Gibbons, P. (2009), Effects of logging on fire regimes in moist forests. Conservation Letters, 2: 271-277. https://doi.org/10.1111/j.1755-263X.2009.00080.x ↩︎
- Gmünder, S., Zollinger, M., Dettling, J. (2020) Biogenic carbon footprint calculator for harvested wood products – Background data & calculations, Quantis Report, https://files.worldwildlife.org/wwfcmsprod/files/Publication/file/8ac6an0ydo_Biogenic_Carbon_Footprint_Calculator_Methodological_Report_July2020_Quantis.pdf?_ga=2.152971001.134052892.1698520218-1728913712.1698520217n ↩︎
- Crane E, (2020) Woodlands for climate and nature: A review of woodland planting and management approaches in the UK for climate change mitigation and biodiversity conservation. Report to the RSPB. ↩︎
- Peng, L., Searchinger, T.D., Zionts, J. et al. (2023) The carbon costs of global wood harvests. Nature 620, 110–115. https://doi.org/10.1038/s41586-023-06187-1 ↩︎
- Searchinger, T., Peng, L., Zionts, J., & Waite, R. (2023). The global land squeeze: Managing the growing competition for land. World Resources Institute. https://doi.org/10.46830/wrirpt.20.00042 ↩︎
- Guest, G., Cherubini, F. and Strømman, A.H. (2013), Global Warming Potential of Carbon Dioxide Emissions from Biomass Stored in the Anthroposphere and Used for Bioenergy at End of Life. Journal of Industrial Ecology, 17: 20-30. https://doi.org/10.1111/j.1530-9290.2012.00507.x ↩︎