Part 1: Climate Analysis
Madrid falls into two koppen climate classification zones: a cold semi-arid climate (classification BSk) in the metropolitan area, and a mediterranean, dry-summer climate in the west half of the city (classification Csa).
The main characteristics of this climate are hot, dry summers and cold, humid winters. There are also large temperate variations between daytime and nighttime. Importantly, Madrid experiences one of the worst urban heat island (UHI) effects in the world. As a result of these conditions, successful ecological design for Madrid will require elements for the regulation of temperature year-round. It will also require strategies to improve the Outdoor Thermal Comfort (OTC) by using materials with high thermal mass and incorporate climate responsive design, like shading devices and water features.
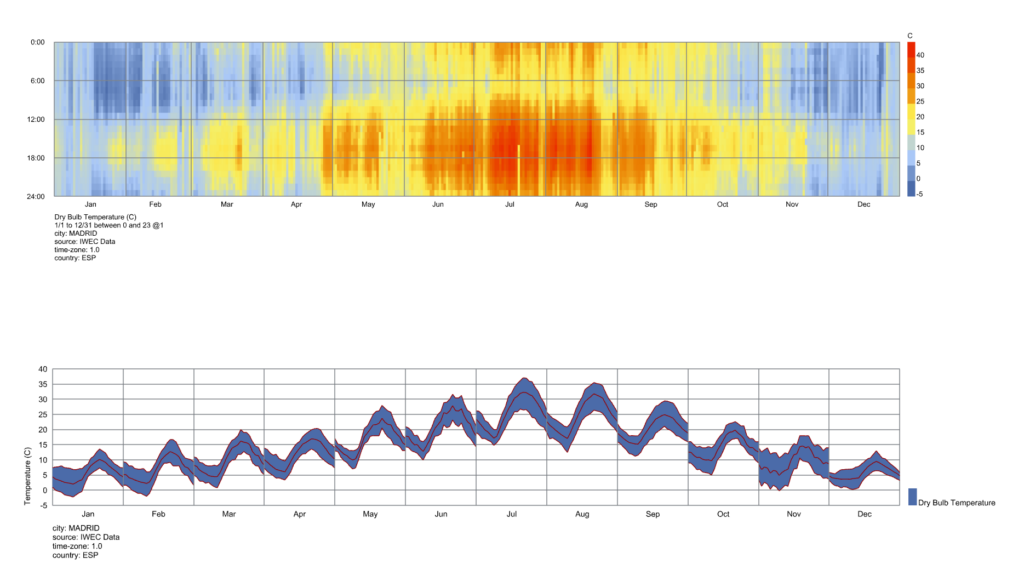
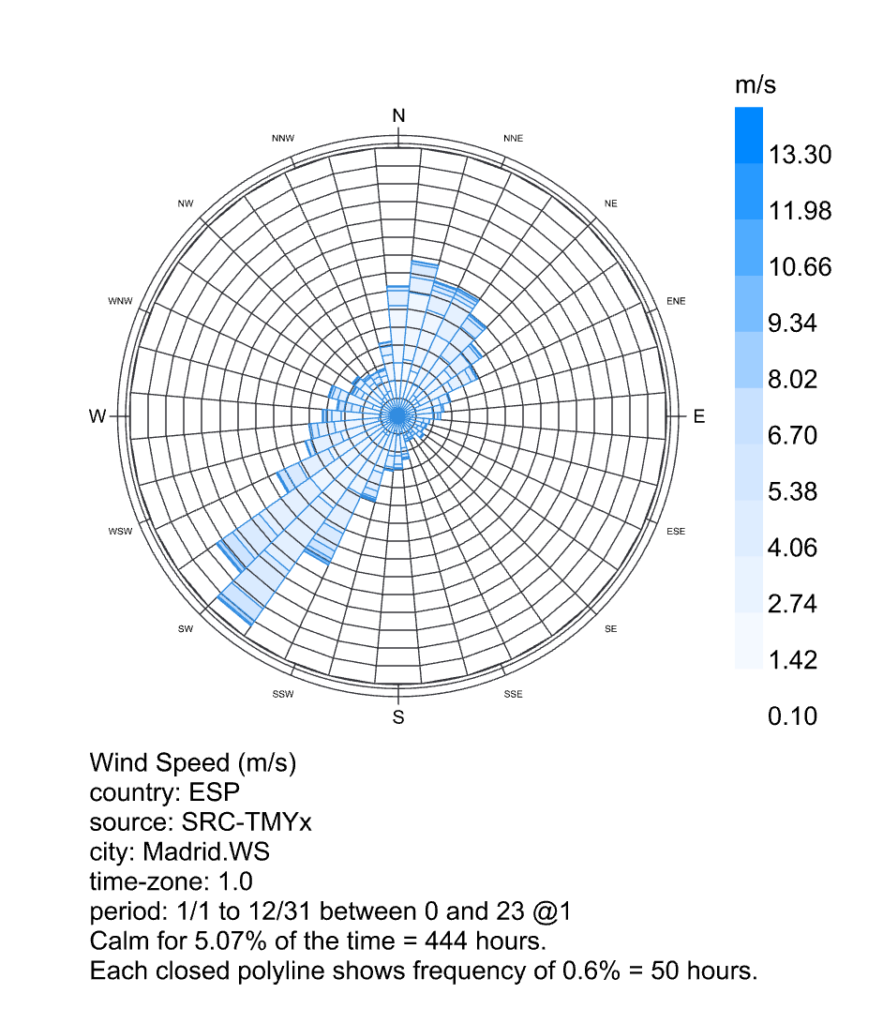
Key metrics
Highest temperature: 35.5°C
Lowest temperature: -11.2°C
Summer average temperature: 25°C
Winter average temperature: 7.3°C
Summer average humidity: 44%
Winter average humidity: 74%
Average wind speed: 2.28m/s
Prevailing direction: 220° (SW)
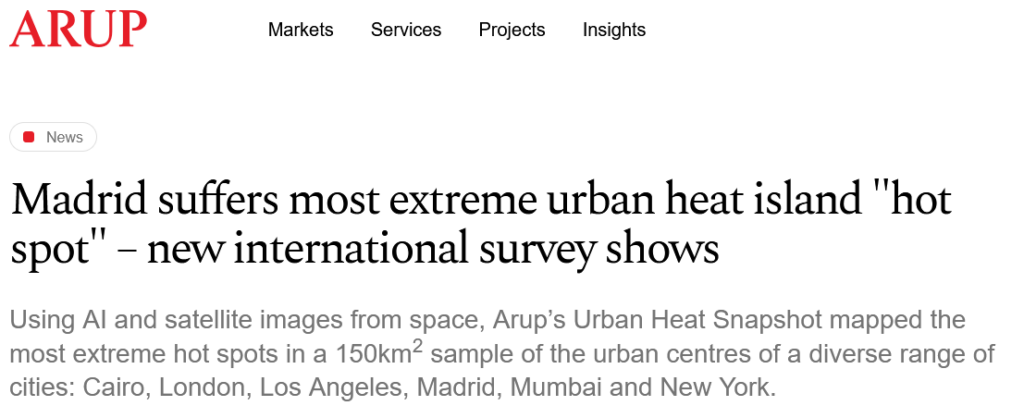
The Urban Heat Island Effect (UHIE) is a phenomenon where urban areas experience significantly higher temperatures than their surrounding rural or suburban areas. In Madrid, the UHIE is getting worse year on year1. The temperatures can differ up to 15 degrees depending on the presence or absence of green areas, vegetation, air flow, the density of buildings, pavements and people. It leads to higher nighttime temperature, higher energy use and health issues. Despite being one of the greenest cities in Europe, the city is also the 4th city in the world2 with the highest deaths due to heatwaves.
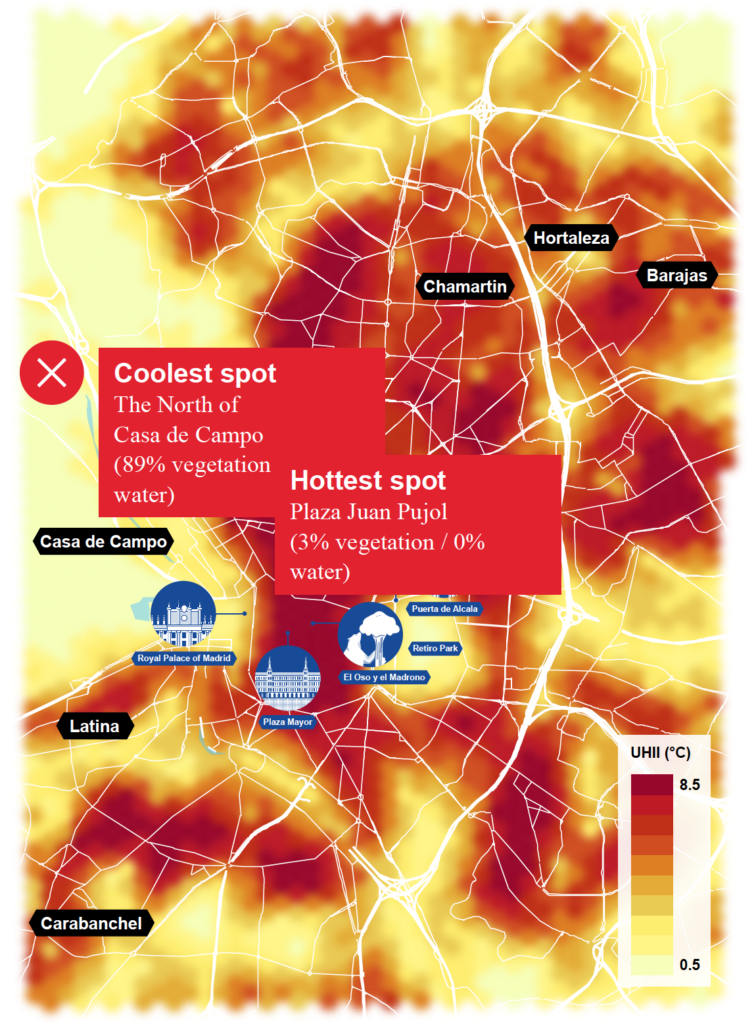
- https://www.arup.com/news/madrid-suffers-most-extreme-urban-heat-island-hot-spot–new-international-survey-shows/
↩︎ - https://interactive.carbonbrief.org/attribution-studies/index.html ↩︎
Part 2: Site and Design Considerations
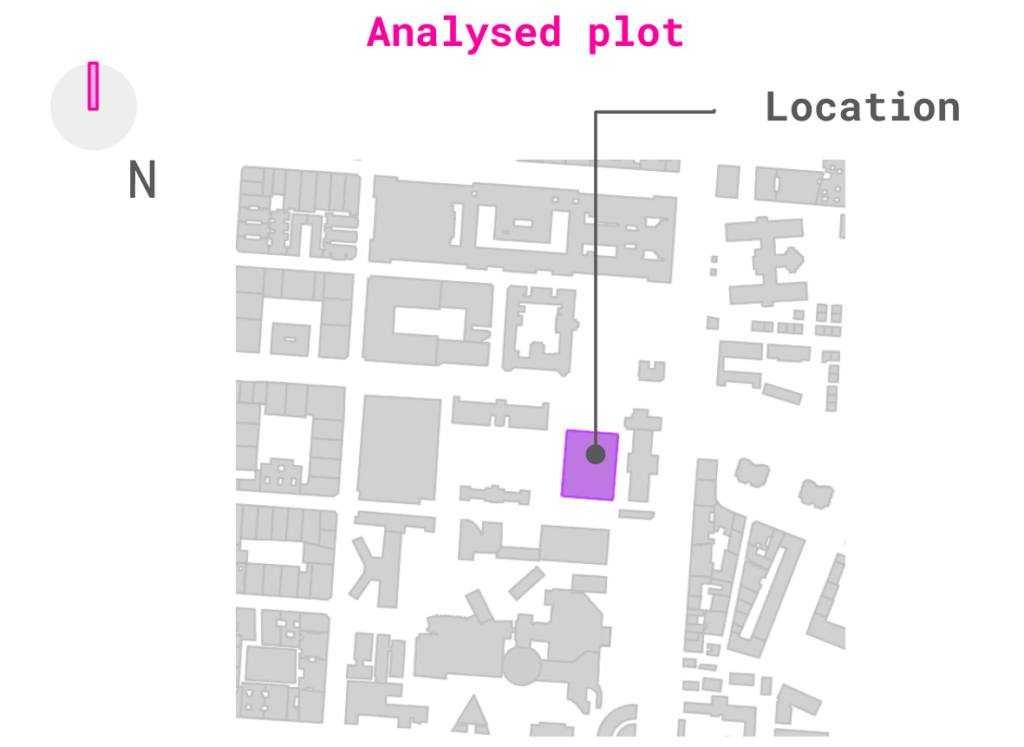
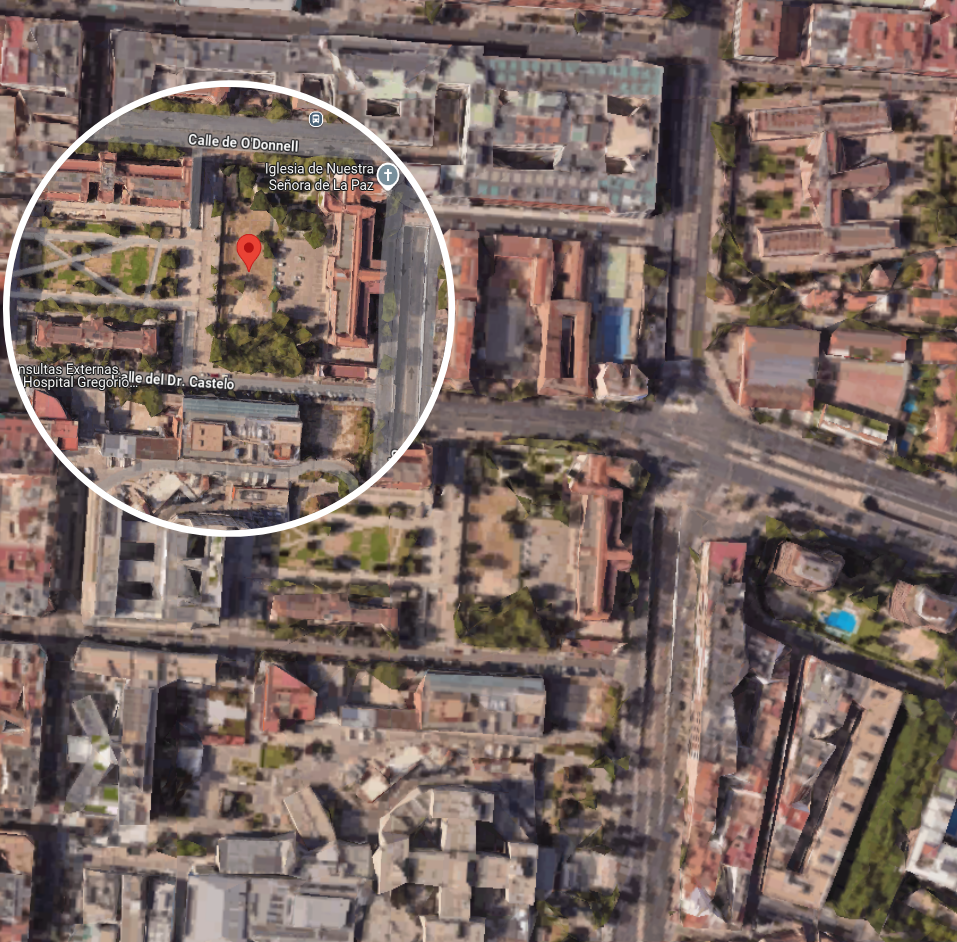
Our chosen location for our site is a medium-high density metropolitan area near O’Donnell metro station. We aim to generate a four-sectional building with variable building levels to analyse our performance criteria, with the goal of analysing the placement, orientation, and dimension of external shading devices for panoramic windows.
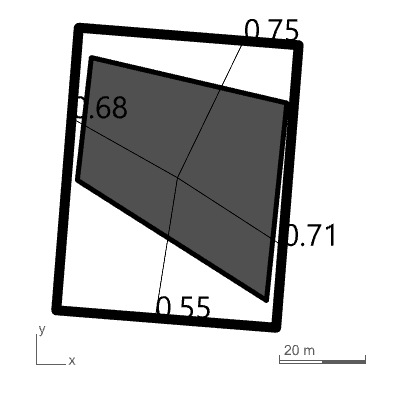
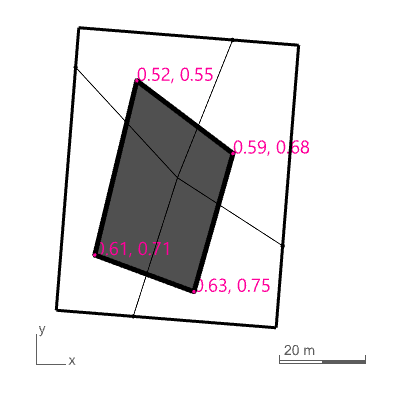
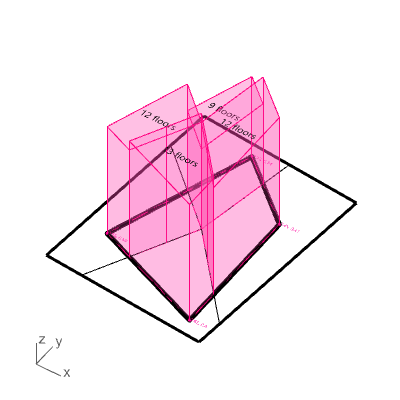
Our design parameters that influence the overall form of the building are the subdivision of the plot into four smaller parcels – we then evaluate surfaces of the four subdivisions to establish the building footprint. Through these two steps we effectively draw the building outline and create the potential for orientation changes when running optimisation algorithms. Finally, we introduce variable building levels for each of the four sections.
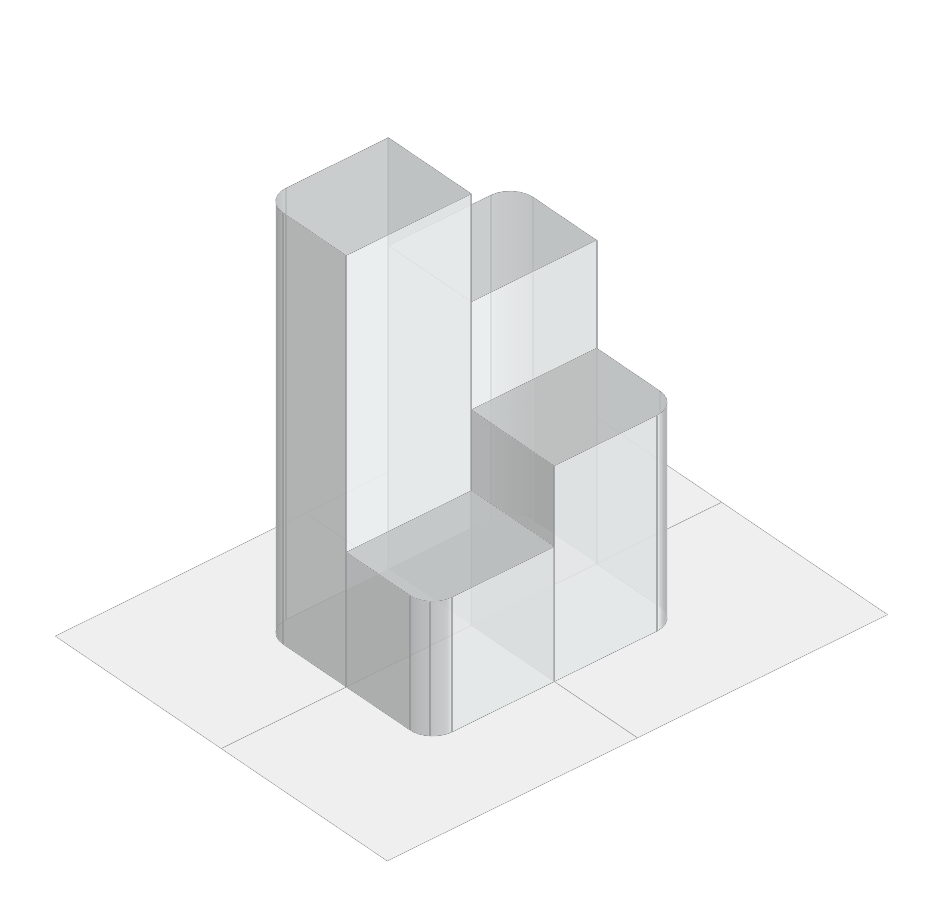
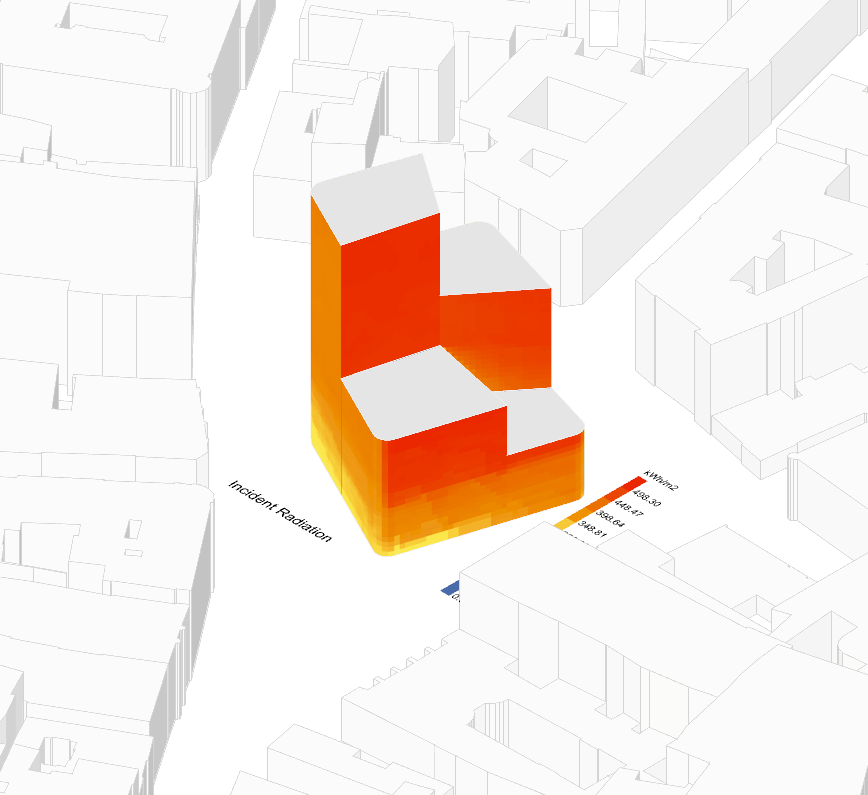
We conducted a multi-objective analysis using Biomorpher to get a design-oriented solution with some floor difference and increased built area. Our optimisation goals were: maximisation of built area, maximisation of floor difference, minimisation of summer radiation, and maximisation of winter sun hours.
The results of our radiation analysis were:
Built area: 13,078sqm
Floor difference (sum): 14
Summer radiance: 1,987,000kWh
Winter sun hours: 3756 hours
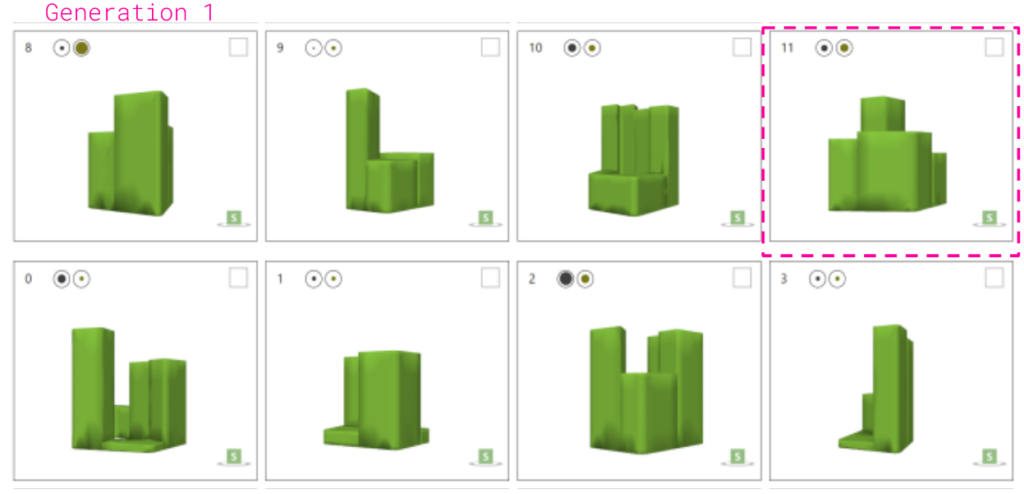
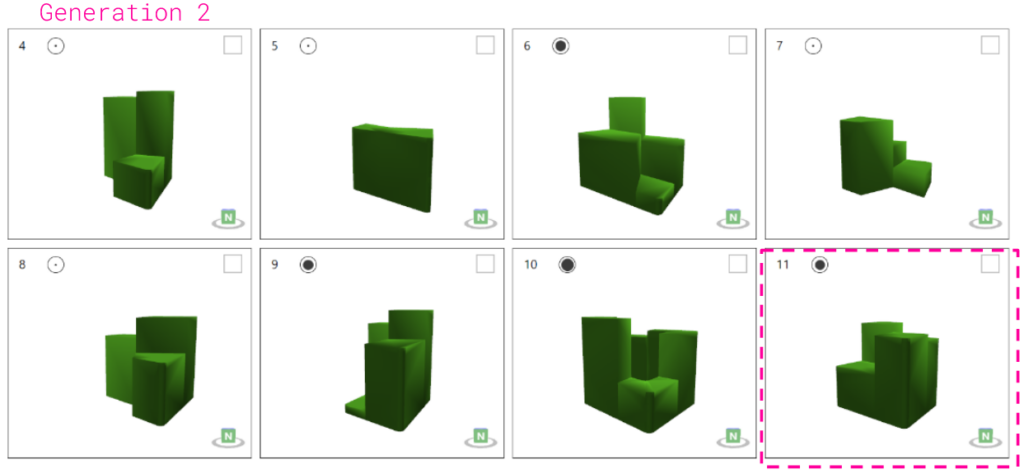
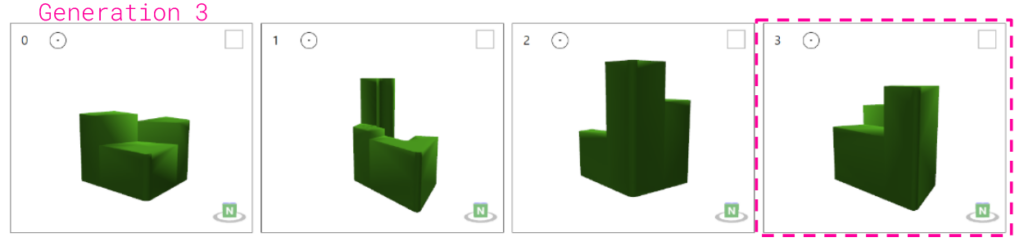
Definition of shading devices
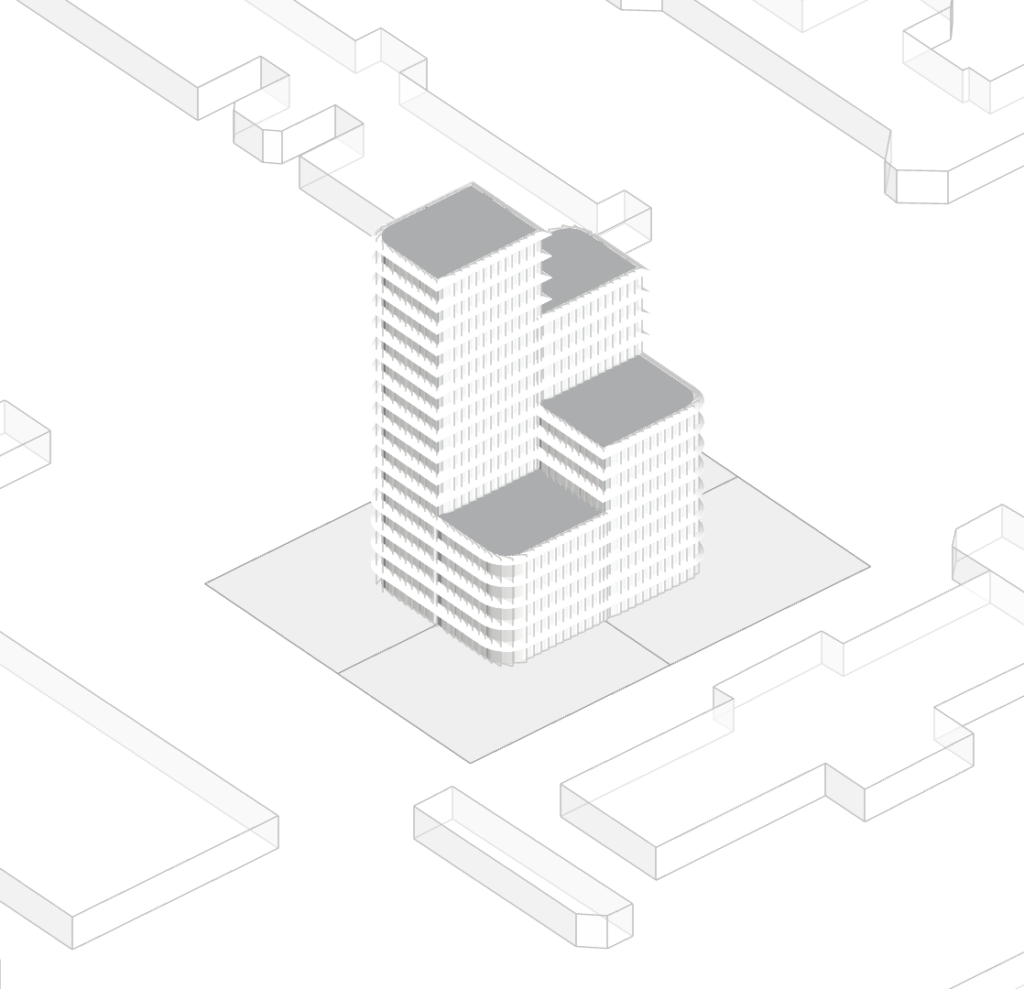
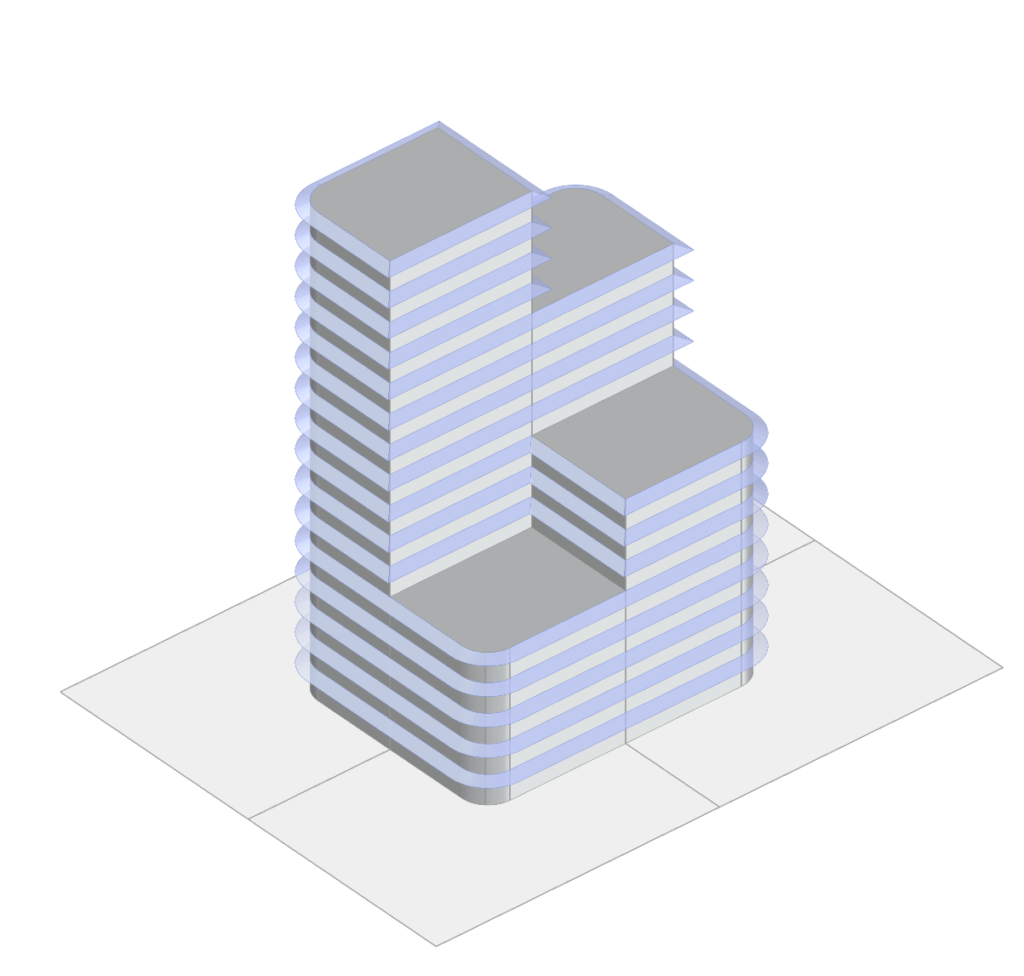
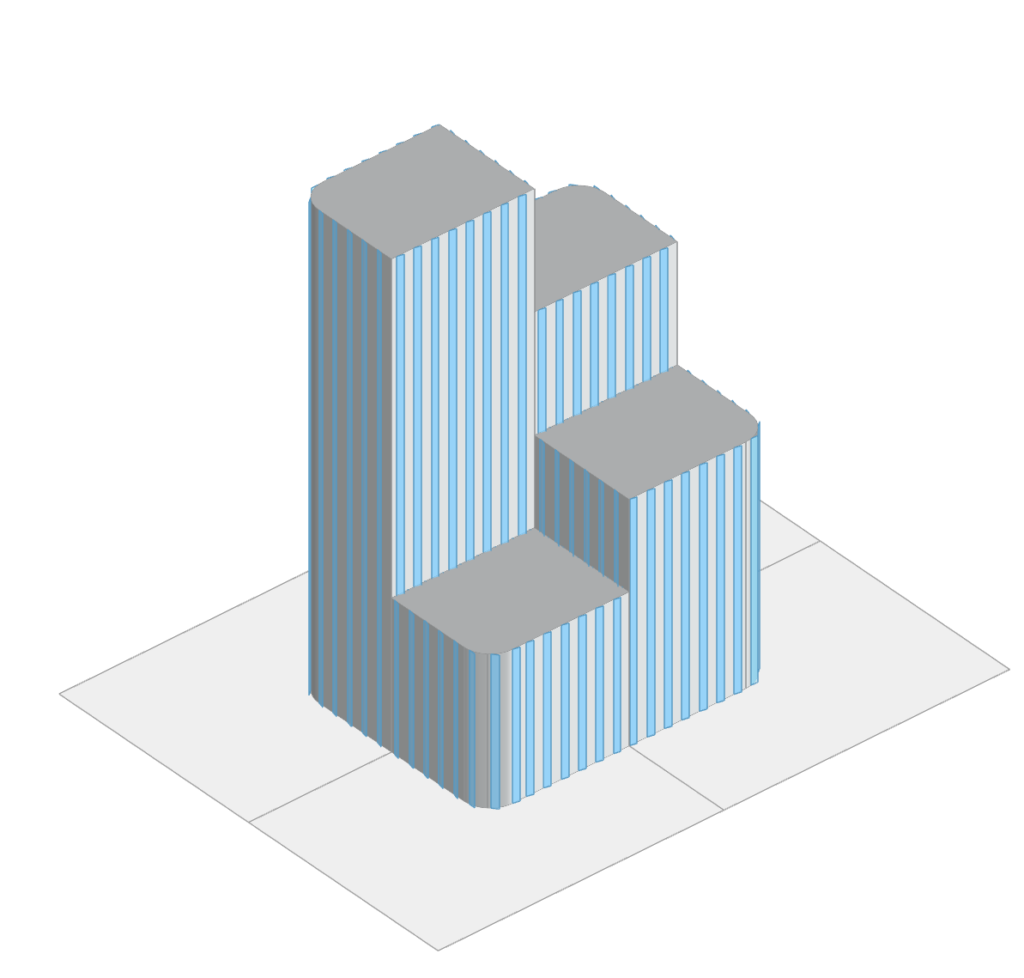
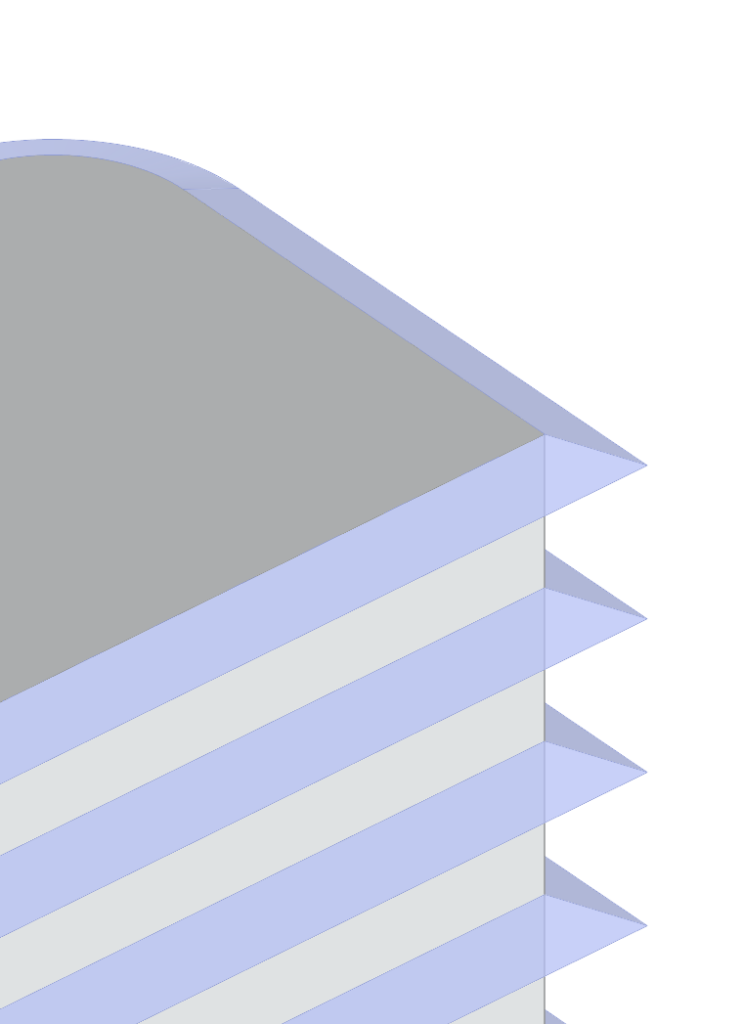
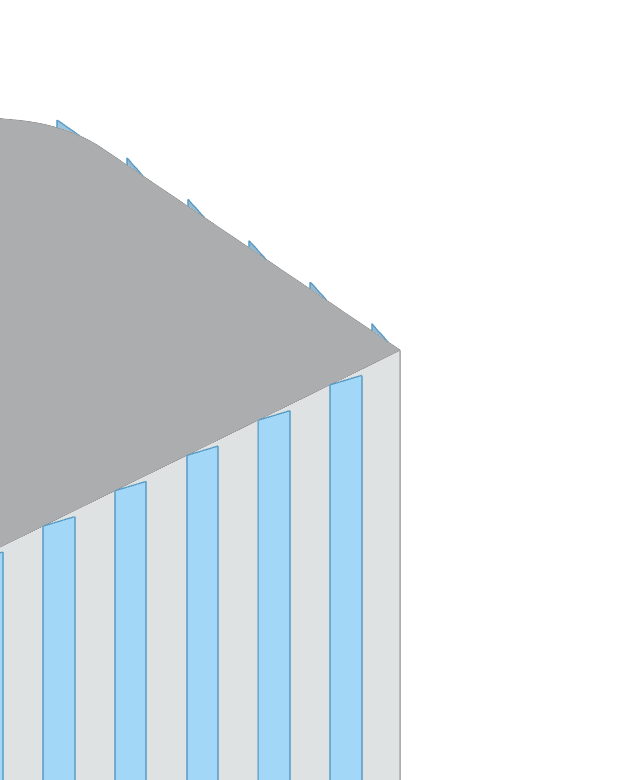
We’ve created 2 types of shades for the 4-sectional multistorey building: vertical and horizontal ones. Each types defined with various parameters, such as shading distance, angle and the distance between the shades.
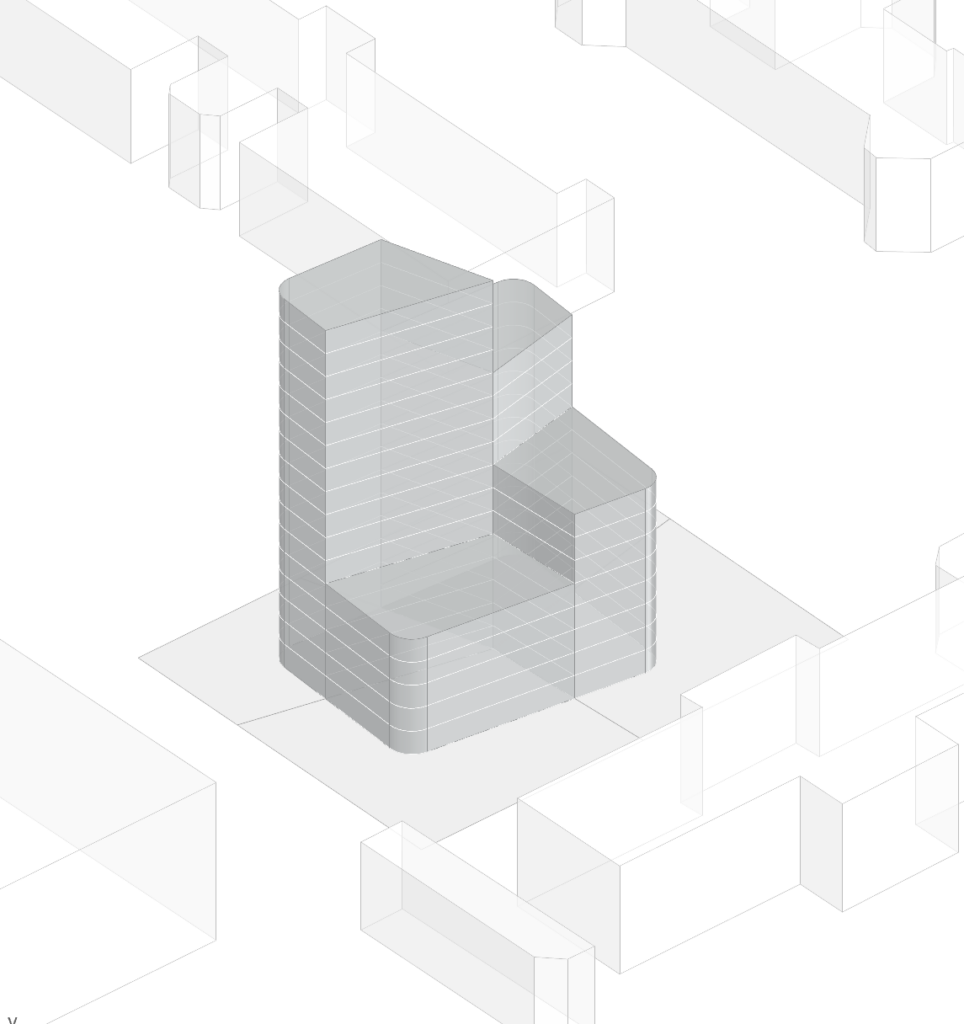
We adapted a building shape and started analysing what type of shades were the most suitable for the summer heat. So, that t would be exposed to less radiance in summer and spend less on air conditioning.
Summer radiation results: 1,987000kWh
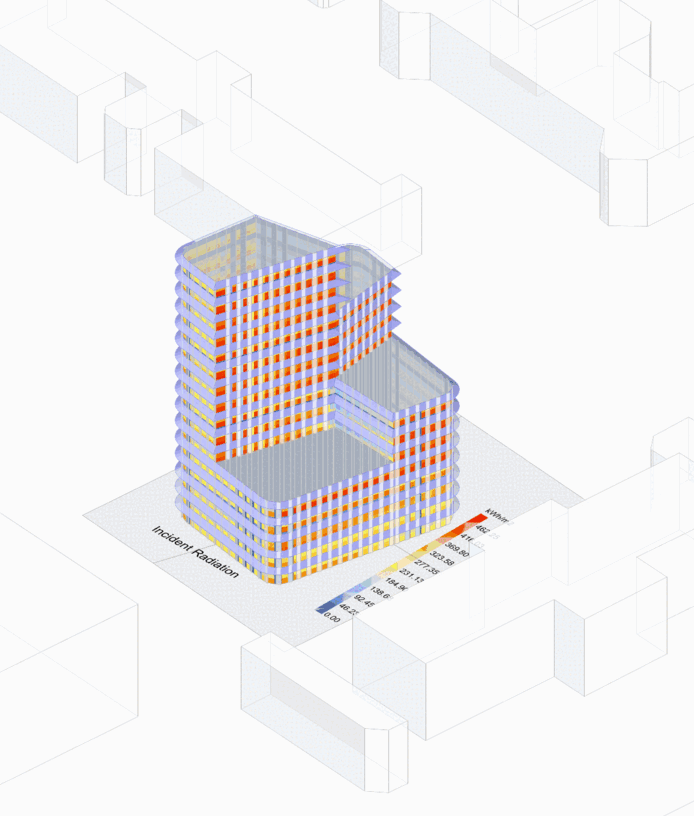
The optimisation was run for finding the best shades positions (both vertical and horizontal) for the building that minimses the incident radiation in summer.
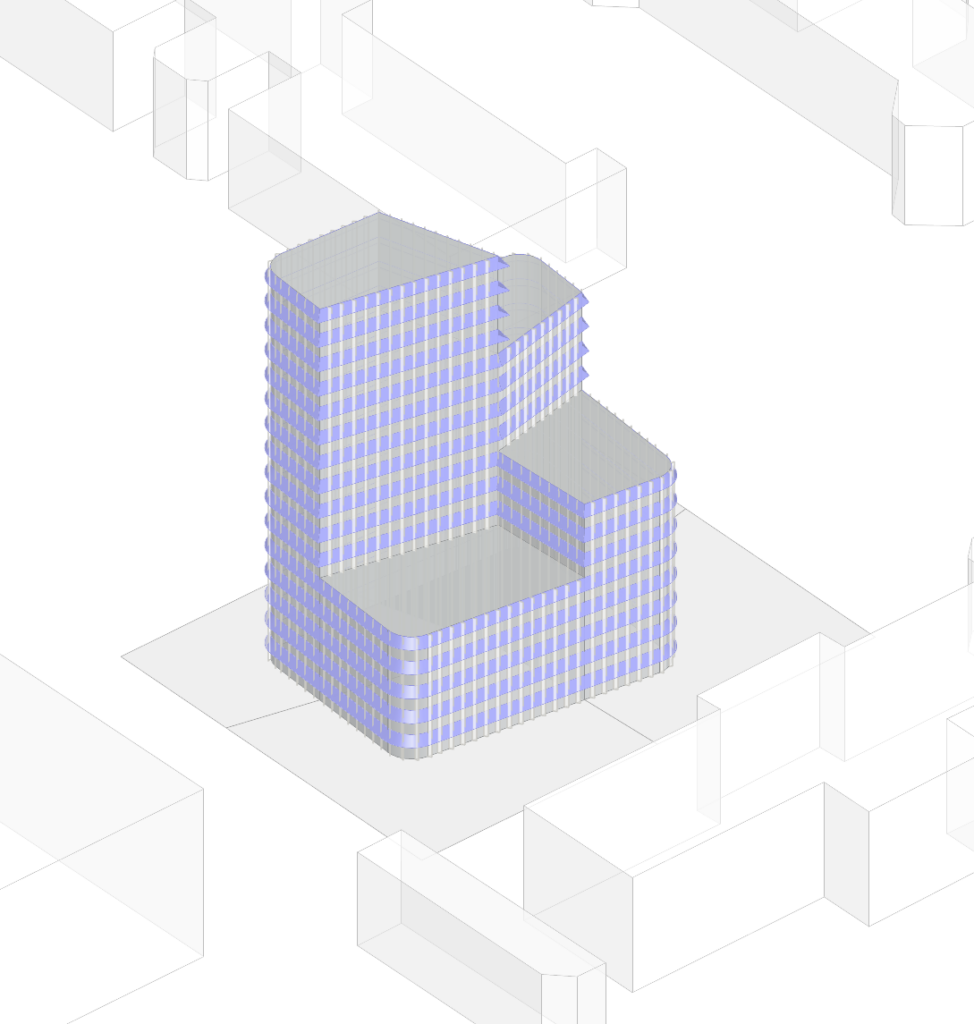
Optimised inputs:
Vertical shading distance: 0.4m
Angle: 13°
Distance between shades: 2.0m
Horizontal shading distance: 1.4m
Angle: 24°
Summer radiation results: 1,362,338kWh
Universal Thermal Comfort Index (UTCI) Considerations
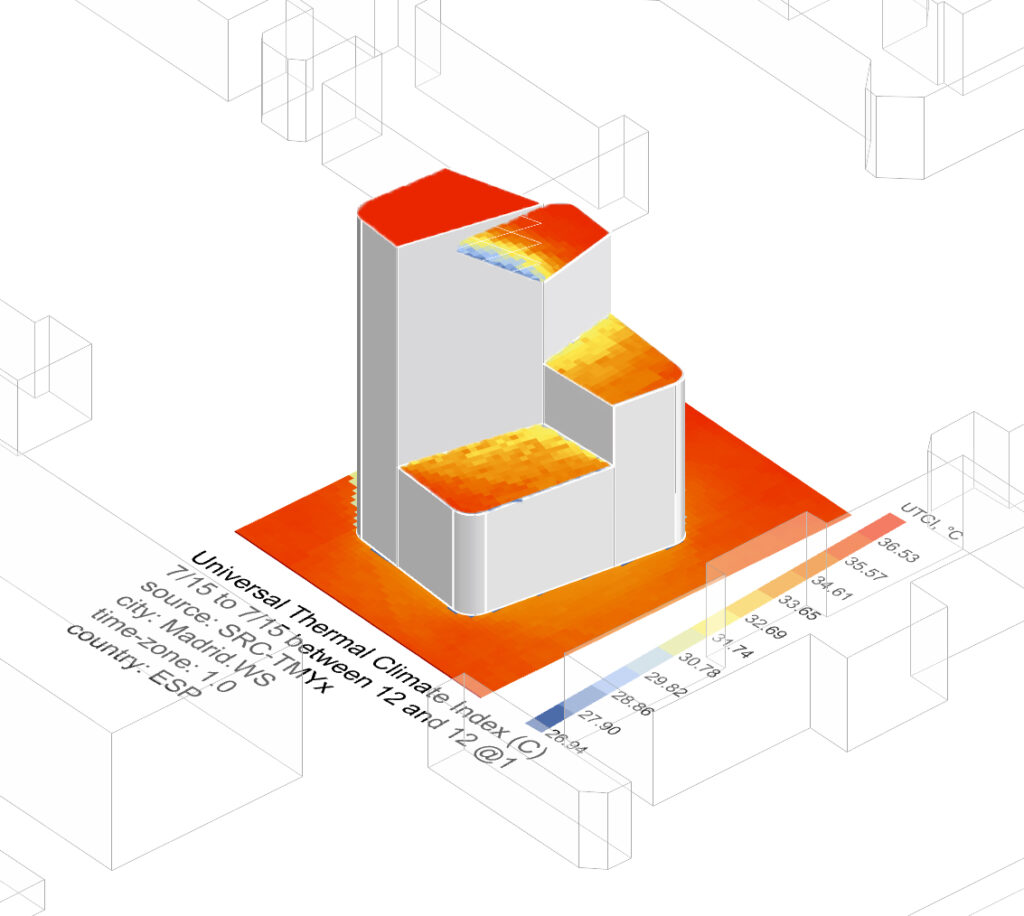
Without any comfort considerations, the ground and roof elements are uncomfortably hot, which increases the interior cooling demand.
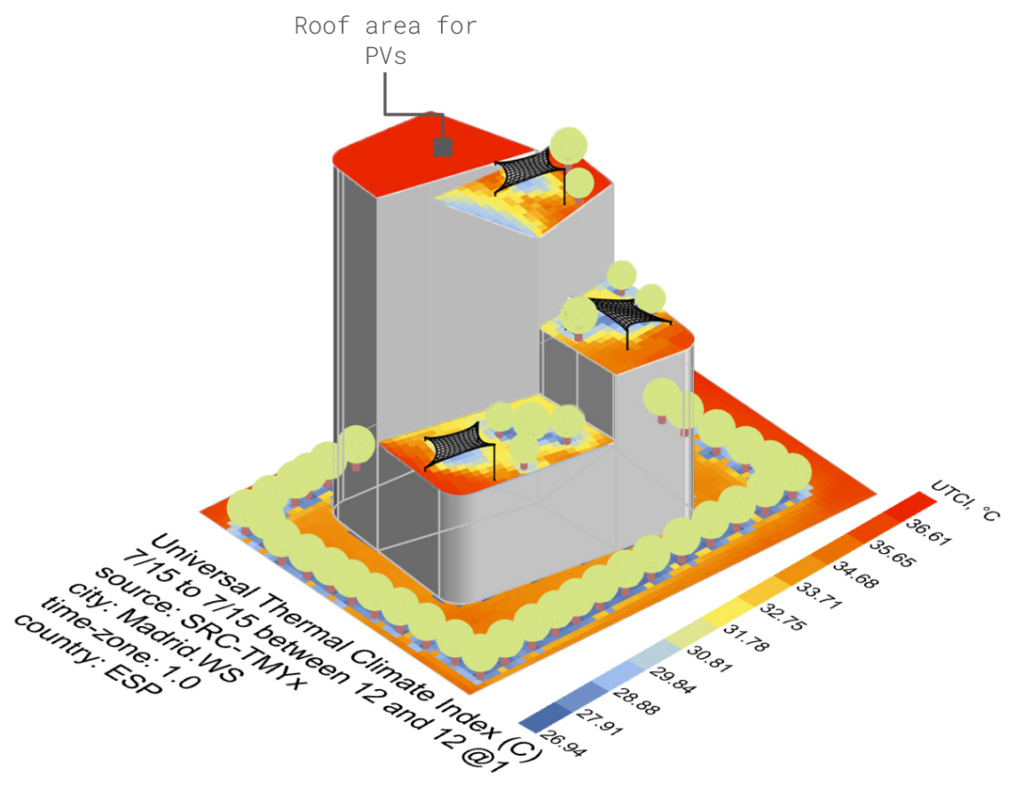
By providing perimeter vegetation coverage, access to and around the building becomes far more comfortable for pedestrians. Rooftop terraces with tensile structures allowes for breakout spaces and reduces interior cooling demand due to roof overheating. A dedicated, uncovered roof area for PVs will also allow for solar energy harvesting.
Daylight Considerations // Daylight Factor vs Sky View
We checked the heatmap of HB Daylight Factor for different types of shades and their angle.
The idea was to find the shades for the panoramic windows that are less obstructive for daylight.
In our iterations, vertical shades seems to be more obstructive than the horizontal ones.
Whereas, the angle haven’t seem to play much role in it for vertical shades.
As the daylight factor analysis has many disadvantages, we checked our suggestion in the alternative one – HB SkyView analysis.
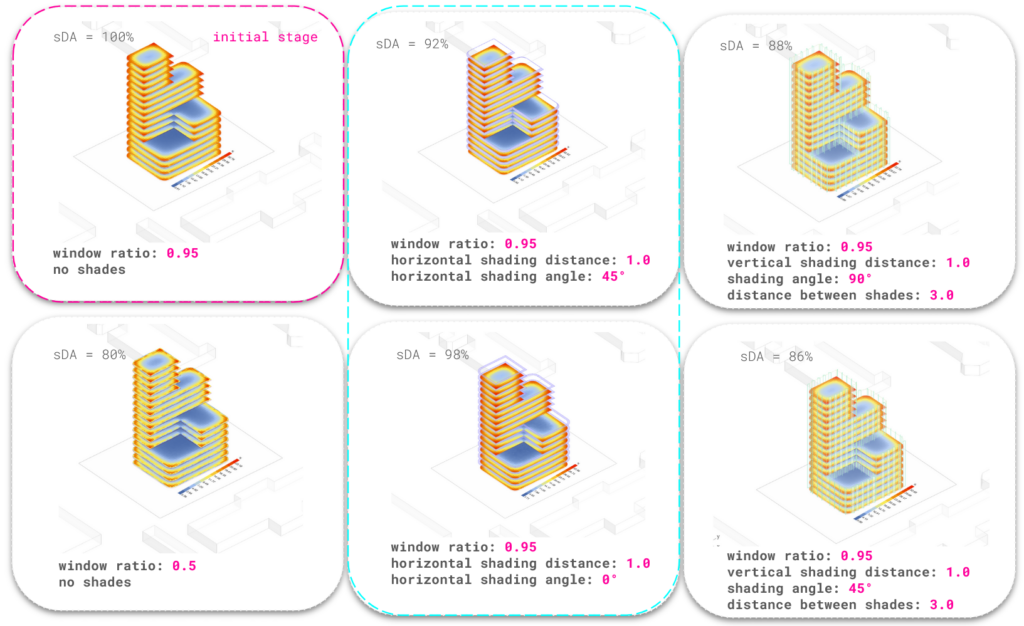
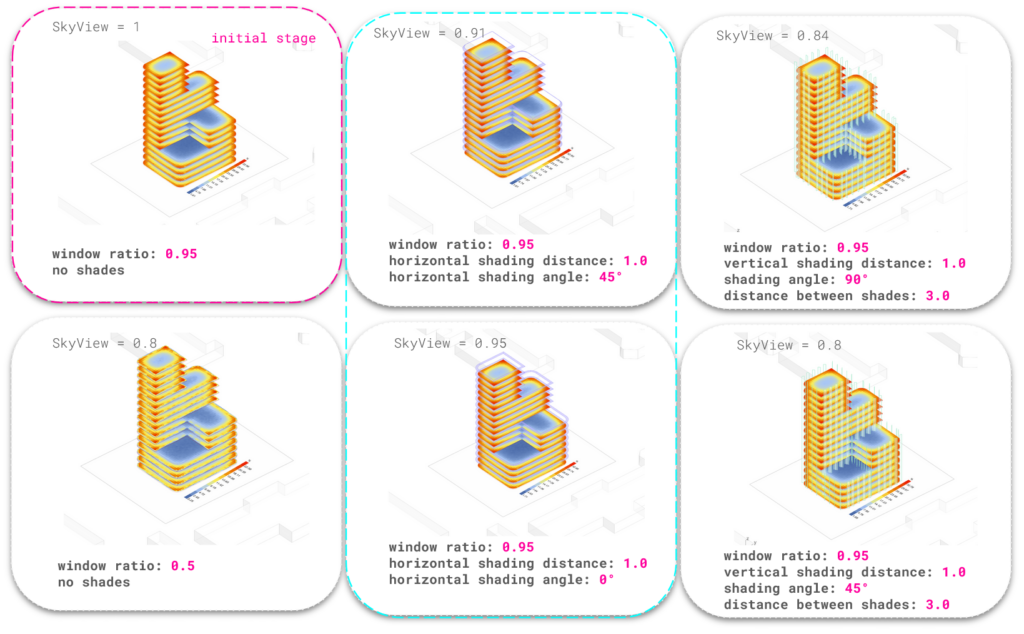
In the alternative method of analysing HB SkyView component for different types of shades and their angles, we noticed the same trend that, for our context, vertical shades are more obstructive in comparison with the horizontal shades. However, the difference between them is slightly lower.
At the same time, vertical shades can be more effective for reducing the cooling demand in summer and heating – in winter.
So, this type of research should be combined with the environmental analysis of the building.
Optimisation toward sDA divided by floor area
By multiplying the % pass rate of spatial daylight autonomy analysis we can optimise toward maximising both the sDA and total floor area.
From this we were able to extract a combination of parameters that yields 100% sDA pass in accordance with IES-LM-83-12 standard for LEED compliance.
Note that for LEED compliance the building must also make use of dynamic interior blinds, however the inputs described on the right are is the shading and height configuration that will make LEED compliance possible as the design progresses.
Much of the 100% pass rate is due to the window ratio of 0.95, the large floor-to-floor height, and the minimal shading elements. In a climate like Madrid, this would be counter intuitive and transfer the impact of the saved operational energy from lighting to cooling demand. As such, a middle-ground solution is always required.
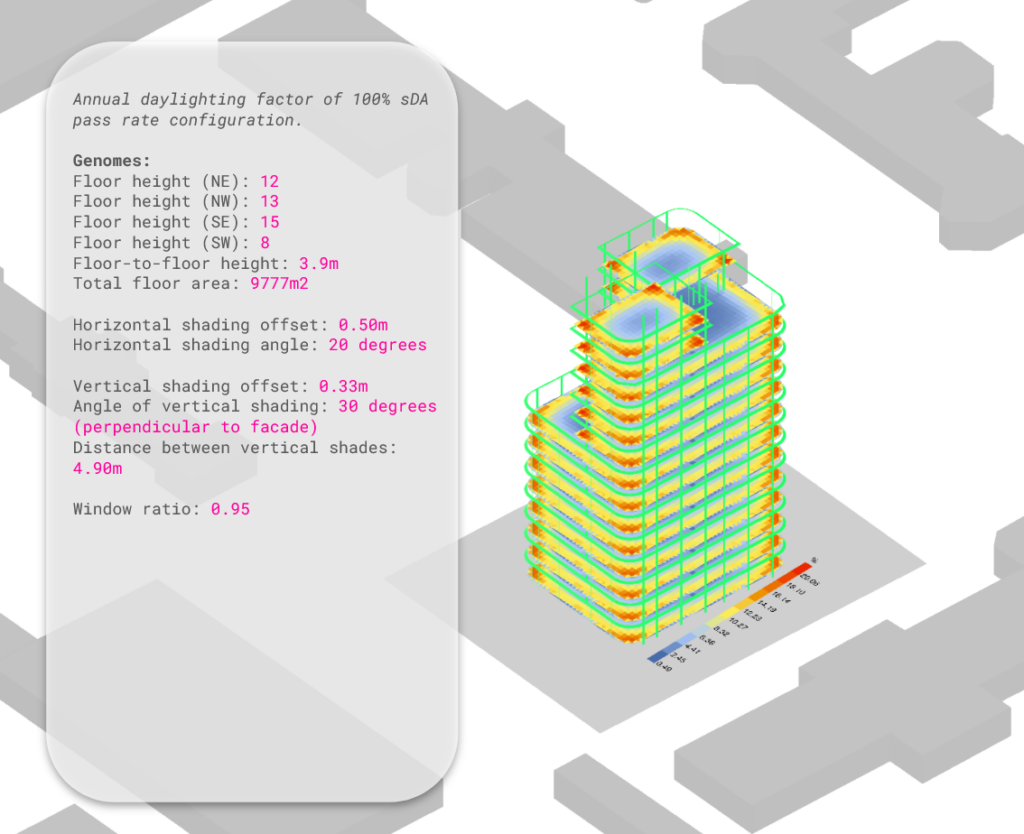
Minimising heat gain while maximising daylight
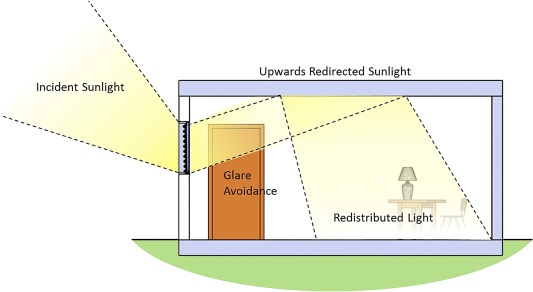
Daylight redirection system: Through the use of prismatic elements in apertures and ceilings, incident sunlight can be redirected from windows and reflecting from the ceiling.
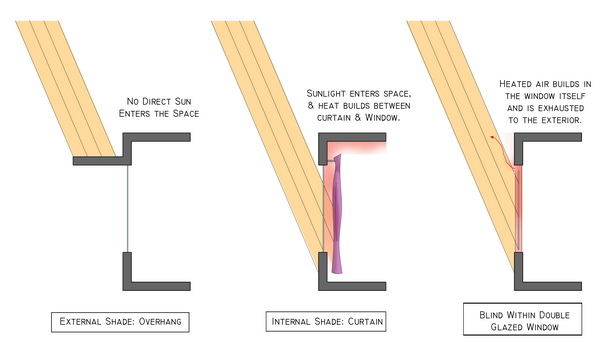
External shading devices: External louvres or other shading devices block indirect radiation before it can penetrate the buildilng and heat intensifies.
Strategic window size and orientation: North facing windows will typically provide more diffuse daylight, while south facing windows will provide consistent direct daylight at the expense of cooling demand. East and west facing windows are subject to low sun angles and are better protected by vertical shading. Deep plan layouts will require larger windows for light to penetrate the interior space, and larger windows will increase cooling demand.
Wind Considerations using Infrared.City
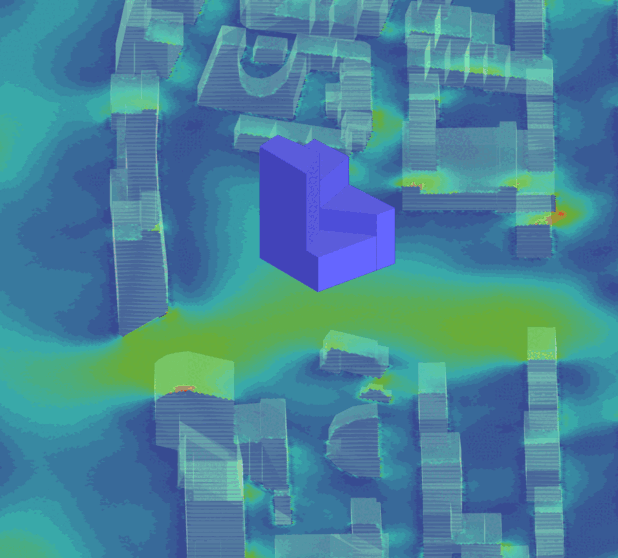
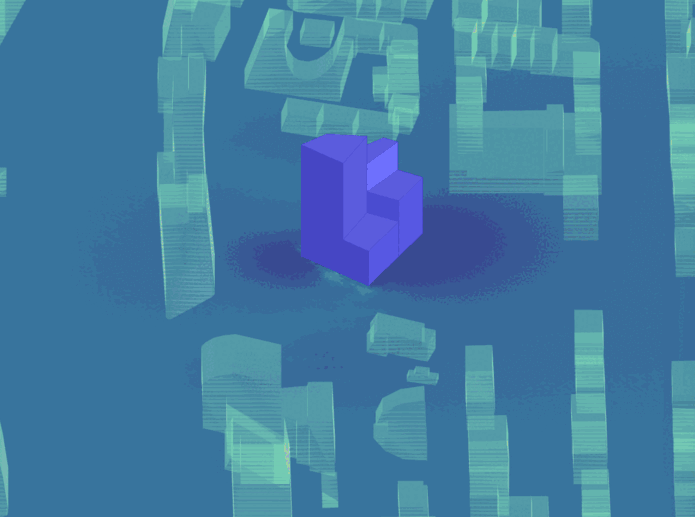
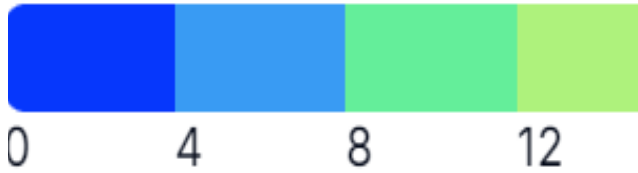
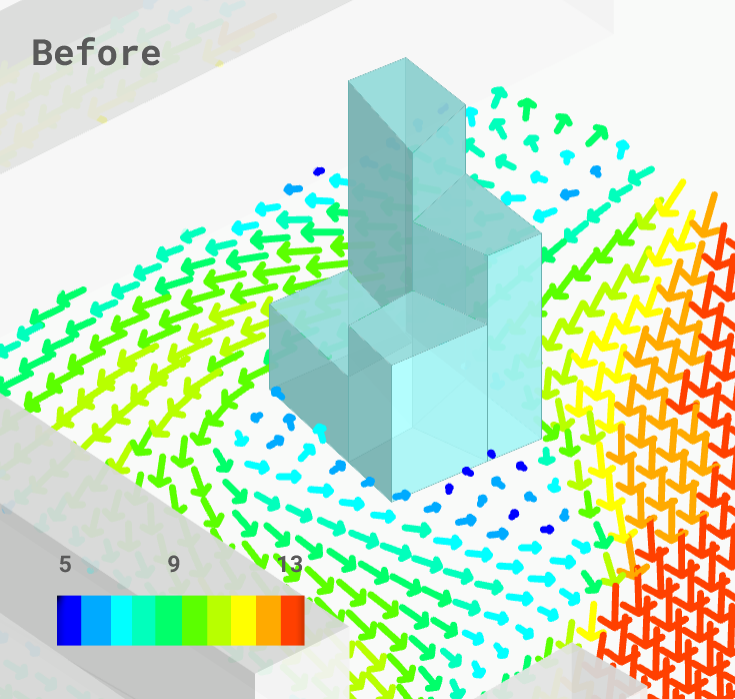
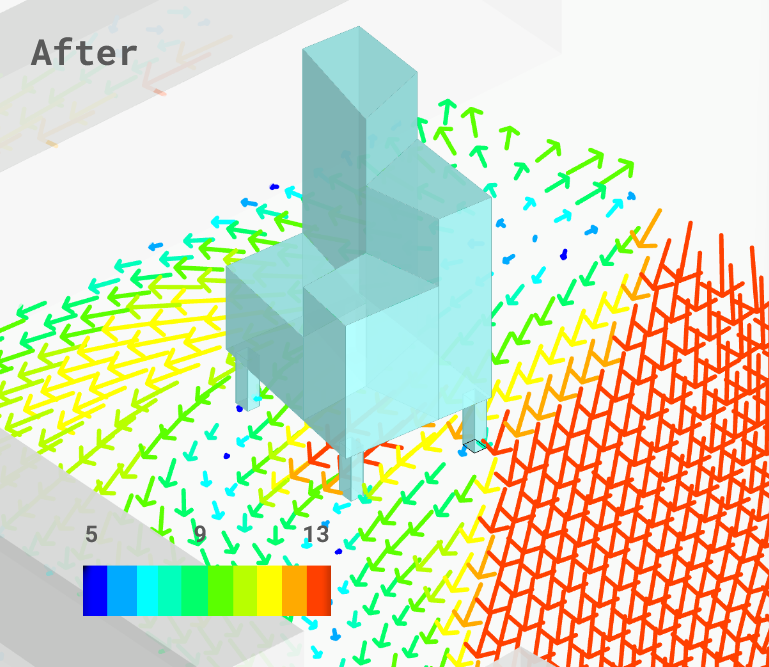
In our simulations, the shape doesn’t affect to the maximum speed value. For the average speed, some insignificant were noticed (by 0.1 – 0.2 m/s only). So, changing the shape doesn’t seem to be reasonable.
As Madrid is experiencing harsh UHIE (Urban Heat Island Effect) during the summer in the city area, we have looked for other solutions – like elevating the building on the legs, so that it will not obstruct the wind and allow it to move freely.
Part 3: Overview of Design Parameters
Taking all of our metrics into consideration, we arrive at a set of parameters that we can change to optimise toward our goals.
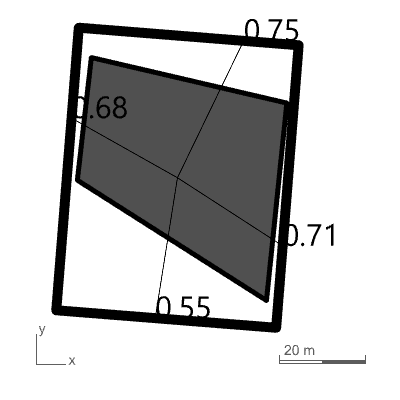
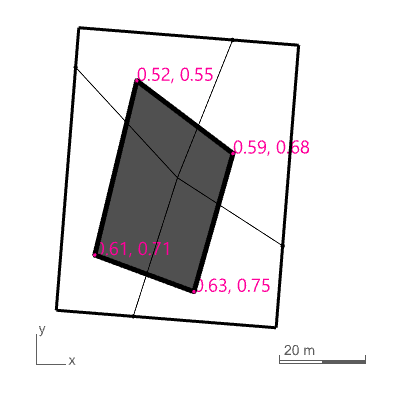
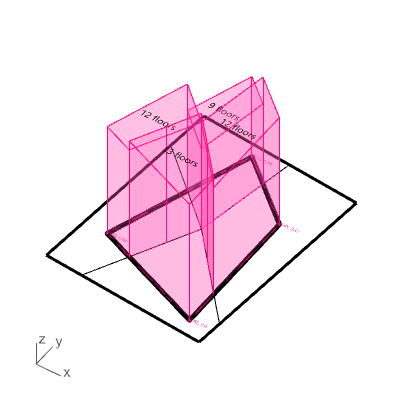
Footprint and levels
Inputs:
Boundary
Levels per section
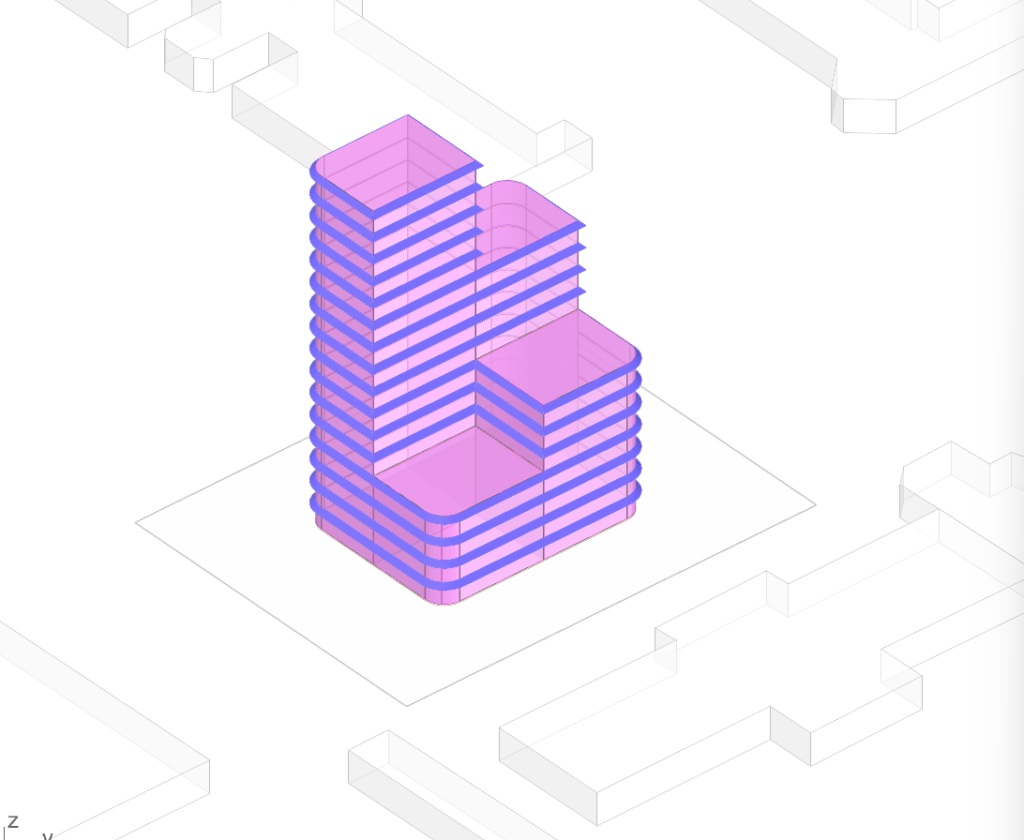
Horizontal shading
Inputs:
Shading distance (m)
Angle of shades (degrees)
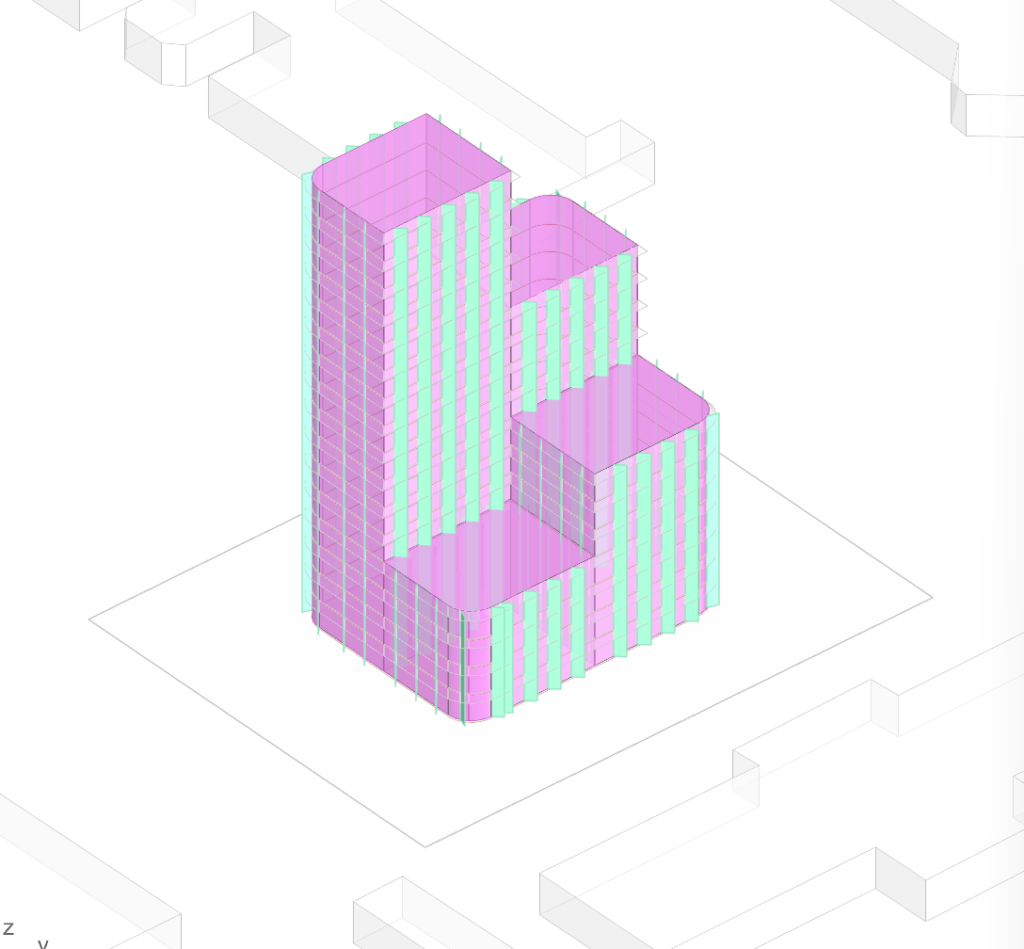
Vertical shading
Inputs:
Shading distance (m)
Angle of shades (degrees)
Distance between shades (m)
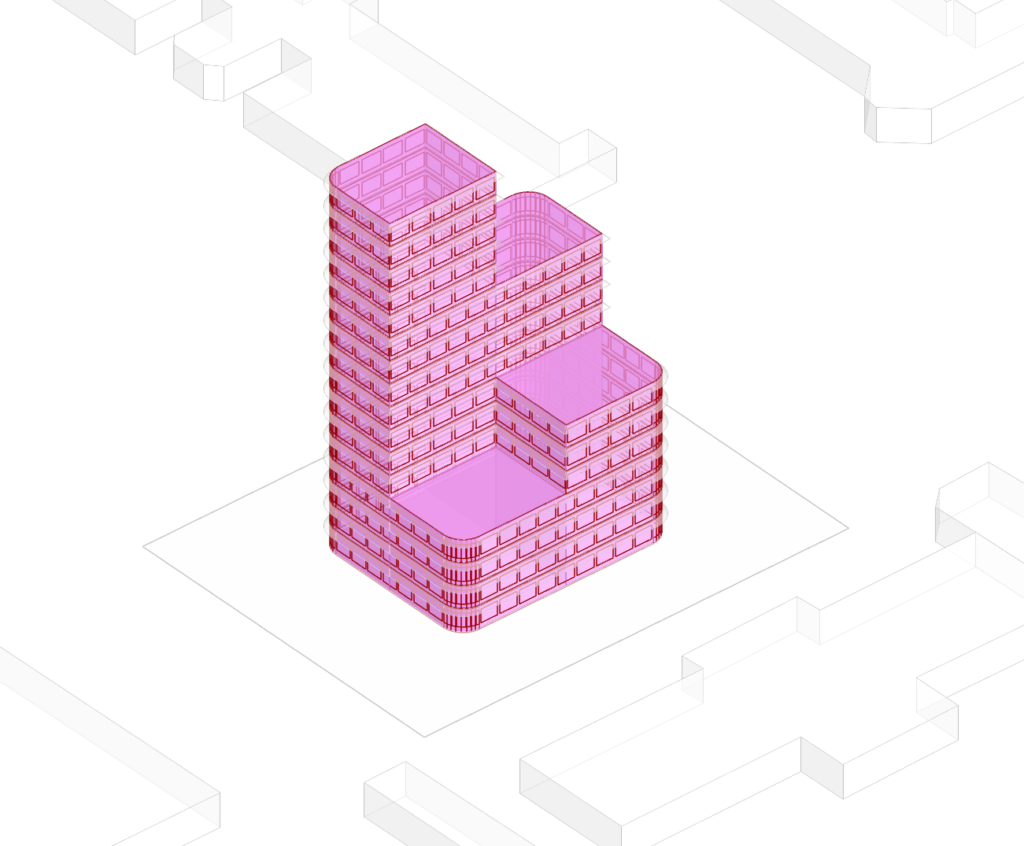
Windows
Inputs:
Aperture ratio
Floor-to-floor height
Design Impact
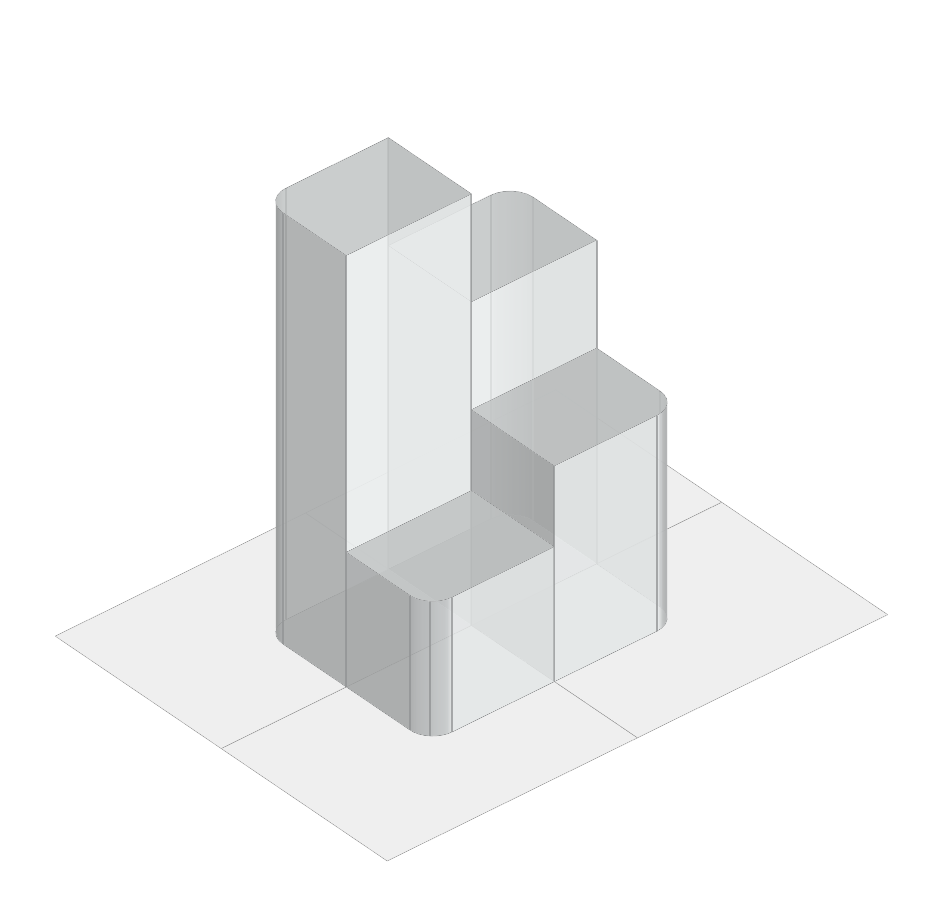
Summer radiation: 2,143,197 kWh
Winter sun hours: 3,197 hours
Wind average: 2.4m/s
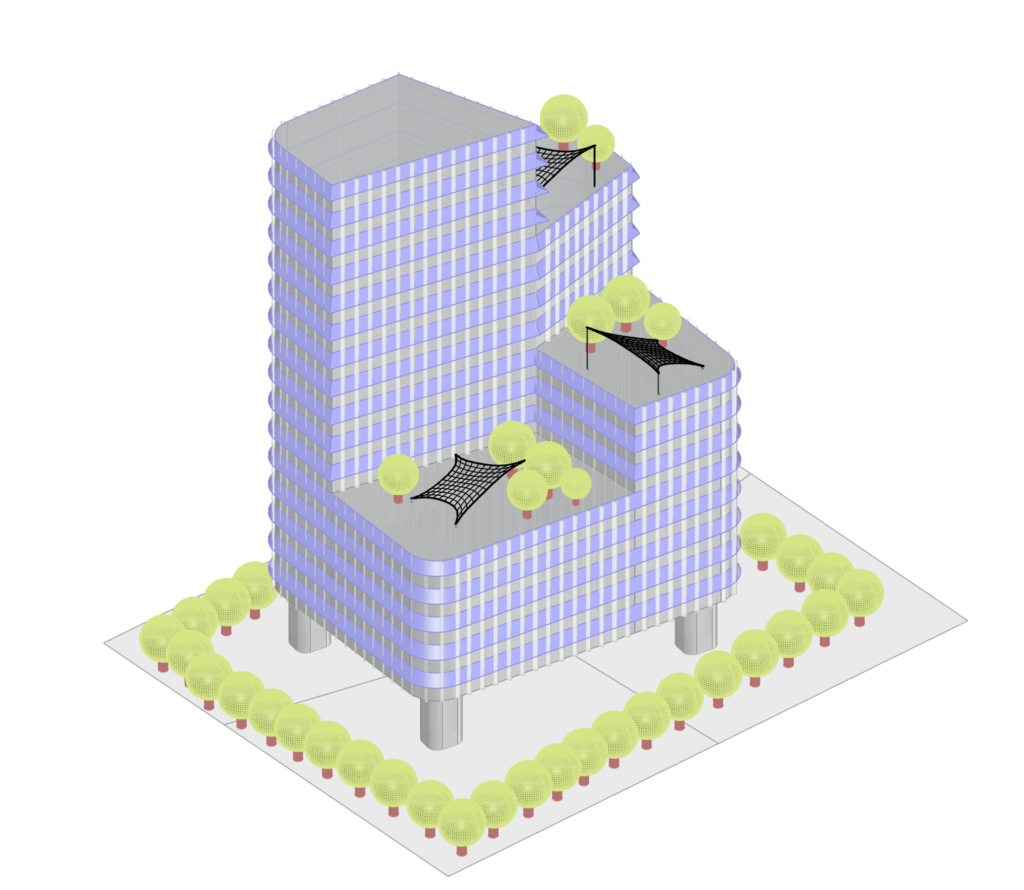
Summer radiation: 1,362,338 kWh (-36%)
Winter sun hours: 3,497 hours (+15%)
Wind average: 3.2 m/s (+33%)
Our adapted building shape saw huge advantages in deploying these environmental tactics – 36% summer radiation reduction, a 15% increase in winter sun hours. We did, however, have an increase in on site wind speed, likely due to building height.
Key Insights
Optimising for one performance metric can have an impact transfer to other metrics. For example, maximising for daylight in an arid climate can cause considerable heat gain and increase the cooling demand.
The goal should not be to optimise toward a combination of goals but rather to find a meaningful compromise between all matrices.
In most situations it is not fruitful to optimise toward all environmental goals as the computation time (particularly when dealing with CFD simulations) combined with the large amount of data needed for an evolutionary solver to function makes optimisation toward all of these goals at once unstable and mostly unfeasible
As a result, computing and adaptation of a design to environmental factors should first choose the environmental strategies that are most beneficial to the site.
Areas for further development
More parametric control that affects our performance metrics would yield a design with greater adaptability.
Interior floor layouts and materials could be used to further refine our indoor thermal comfort analysis.
% vegetation coverage of roof and landscaping elements, courtyards or the inclusion of other wind-mitigating elements would further refine our wind analysis.
Ladybug Tools’ Dragonfly component can simulate and measure urban heat island effects using the Urban Weather Generator (UWG) simulator engine. The resulting urban EPW files can be used to assess the impact of UHI on building energy use, outdoor thermal comfort, and passive survivability.
Simulations of rainwater runoff can effectively measure drought prevention strategies.
Further, design considerations such as rainwater harvesting, greywater recycling and drought tolerant landscaping will help to resist drought in summer.