Introduction
In a world where natural resources are increasingly scarce, the construction industry faces a crucial challenge: reducing material waste and maximizing the use of available resources. Wood, a traditionally used construction material, is at the center of this issue. Every year, millions of tons of wood are discarded or underutilized due to its irregular shape, wasting not only material but also the energy and labor involved in its production. In this article, we explore how a new perspective can transform the way we utilize this natural resource.
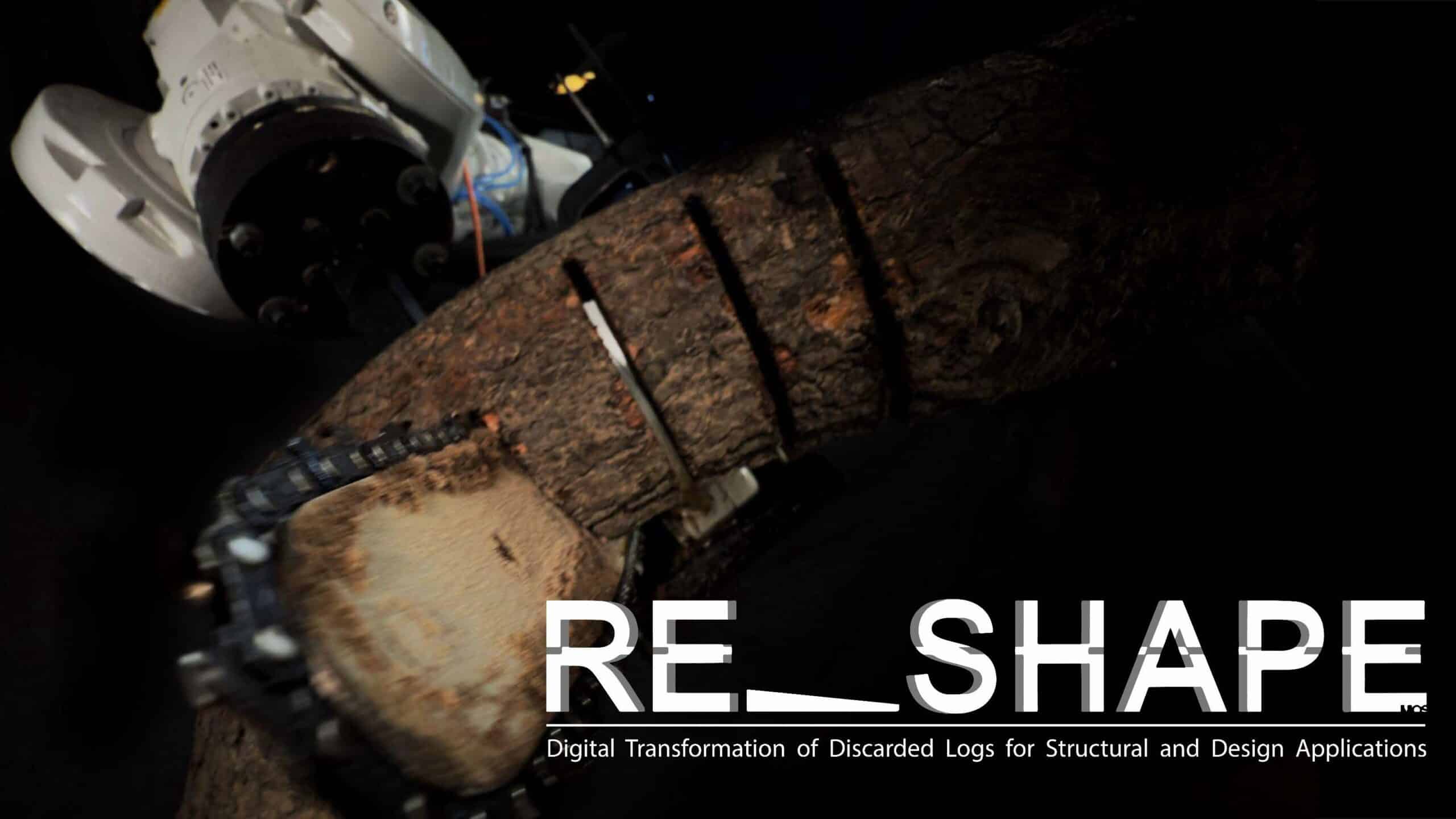
Wood / Waste
Every year, large volumes of logs are discarded due to their irregular shape. Industry data indicates that between 40% and 60% of harvested wood ends up being wasted or downgraded to lower-value products, such as pallets, firewood, or biomass.
In 2017, Spain reported over 1.2 million tons of discarded wood, while in the United Kingdom and Italy, the figures rose to 4.4 and 4.5 million tons, respectively. At a European level, it is estimated that more than 50 million tons of wood are wasted annually, representing a significant economic and environmental loss.
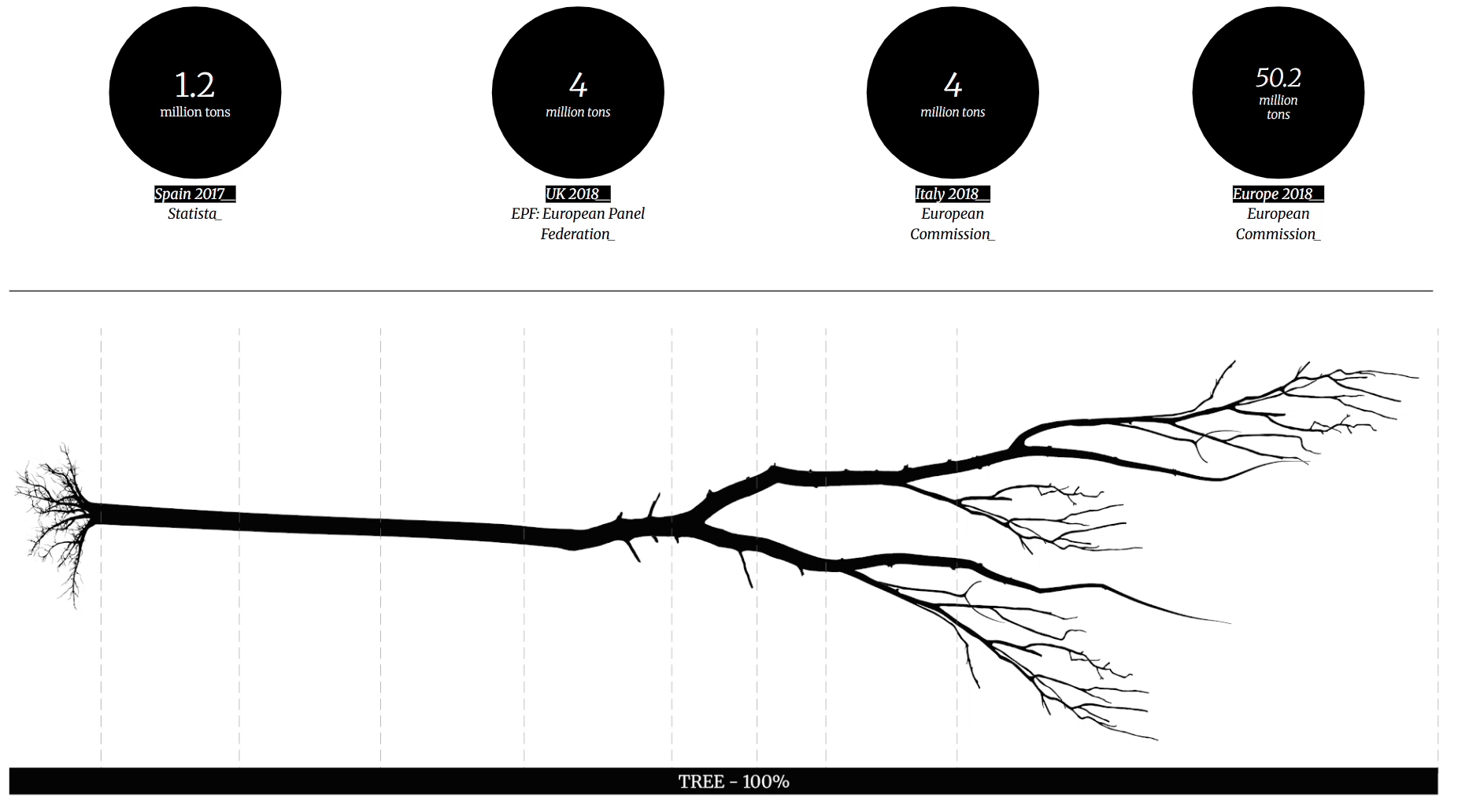
In Catalonia, approximately 64% of the territory is covered by forests, making it one of the most wooded regions in Southern Europe. Predominant tree species include pine, holm oak, and cork oak, which have adapted to the Mediterranean climate over centuries. This extensive forest coverage not only shapes the landscape but also plays a crucial role in the local economy, supporting industries such as timber production, cork harvesting, and sustainable forestry management. Beyond economic benefits, Catalonia’s forests contribute to biodiversity conservation, carbon sequestration, and climate regulation while also providing essential habitats for wildlife and protecting against soil erosion. The responsible use of these resources, combined with technological advancements in digital fabrication and computational design, presents an opportunity to optimize material usage, minimize waste, and create innovative, sustainable architectural solutions that balance economic growth with environmental preservation.
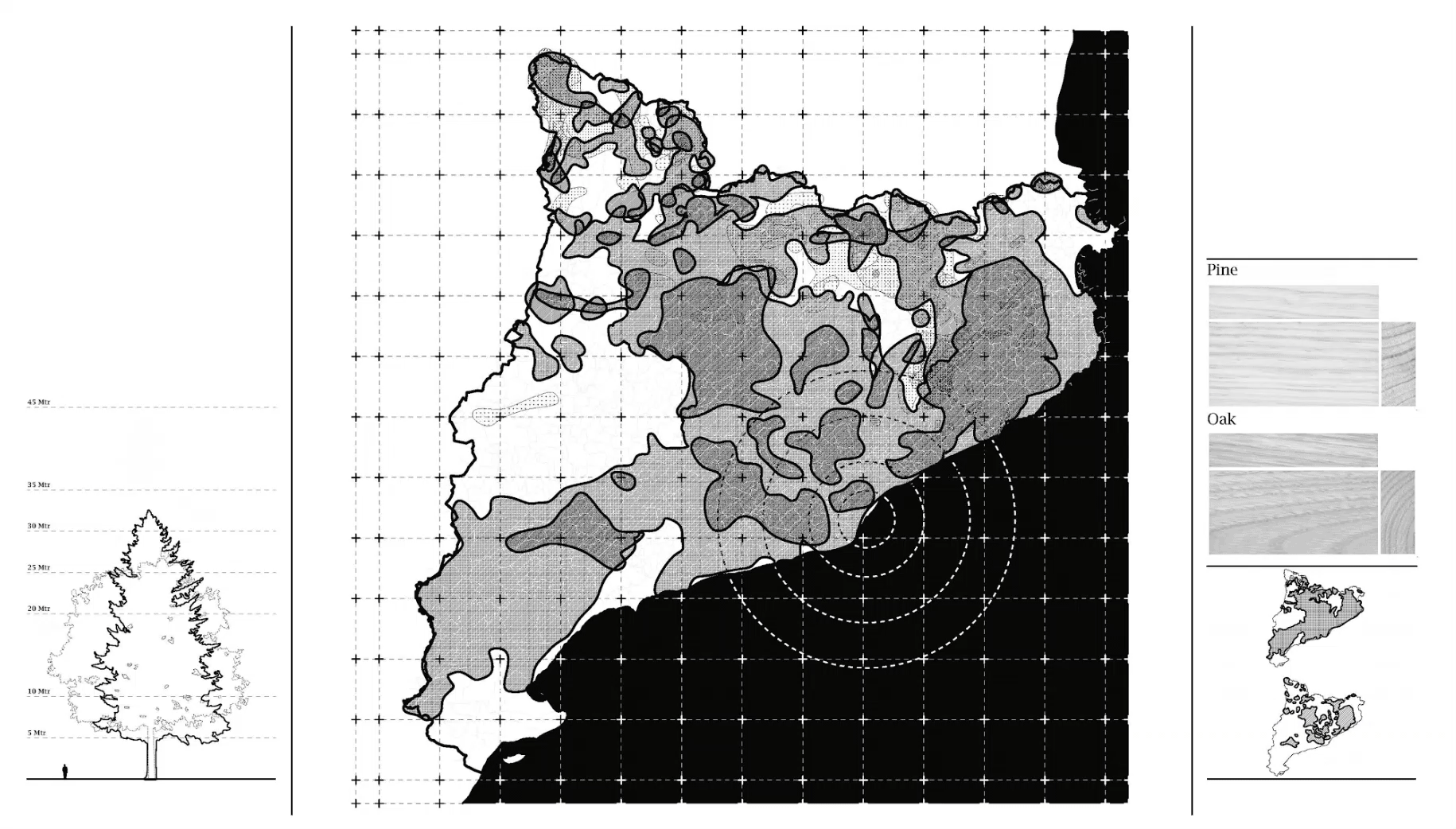
State of Art : Context
In recent years, timber construction has undergone a significant transformation thanks to digitalisation and computational optimisation. Traditionally, the timber industry has been constrained by production methods that favour standardisation and material waste. However, advances in technologies such as 3D scanning, parametric design and robotic manufacturing have enabled a more efficient and sustainable approach, where each piece of wood can be utilised according to its natural characteristics rather than discarded for its irregularity.
This paradigm shift aligns with global trends in architecture and design that seek to reduce the ecological footprint of construction. Innovative initiatives in Europe and other parts of the world have demonstrated how the integration of digital tools in wood processing not only optimises the use of wood, but also opens up new formal and structural possibilities. The combination of natural materials with advanced manufacturing processes marks a new path towards more sustainable, efficient construction aligned with the principles of the circular economy.
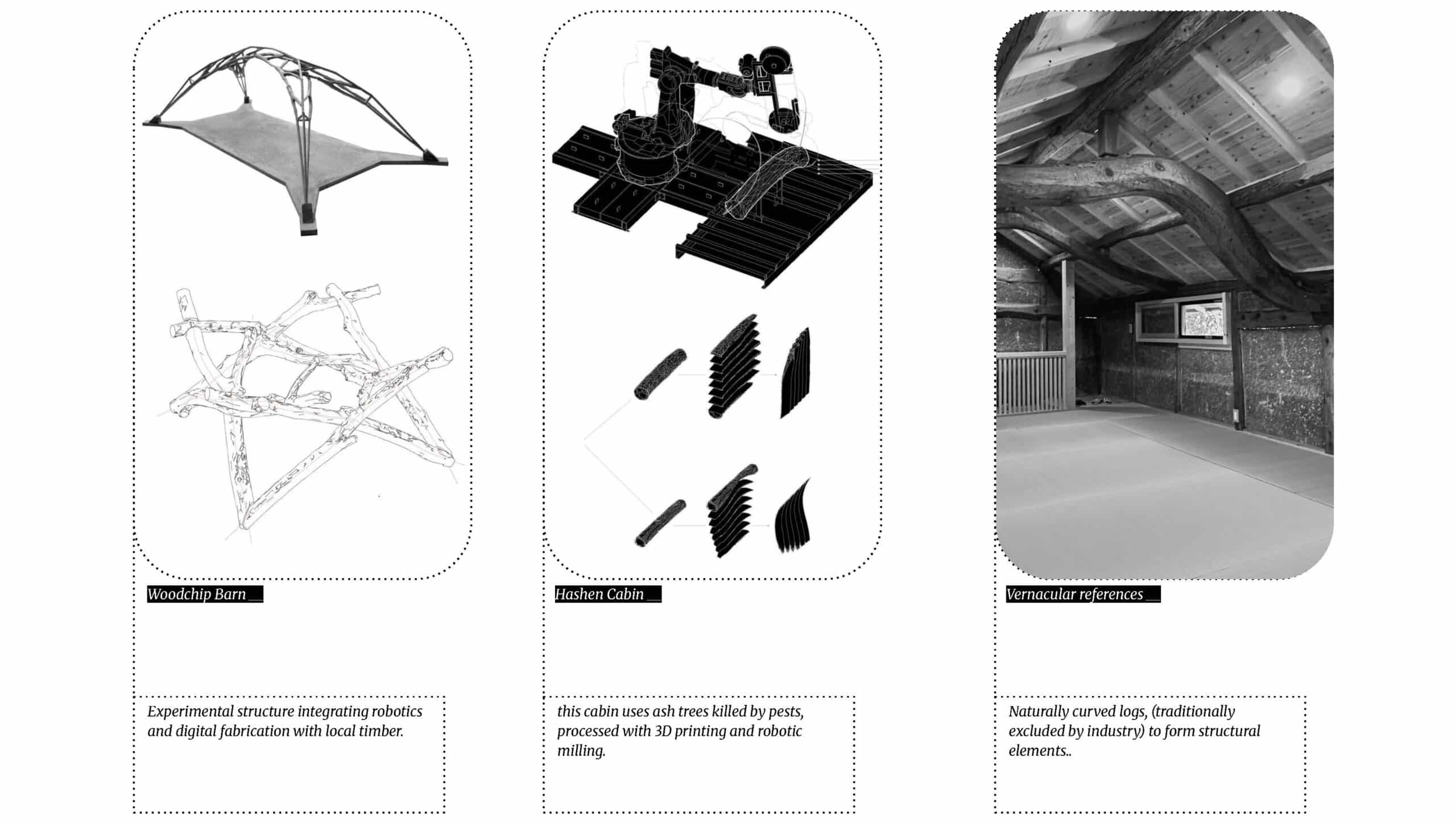
“ The design adapts to the log shape and not the log shape to the design ”
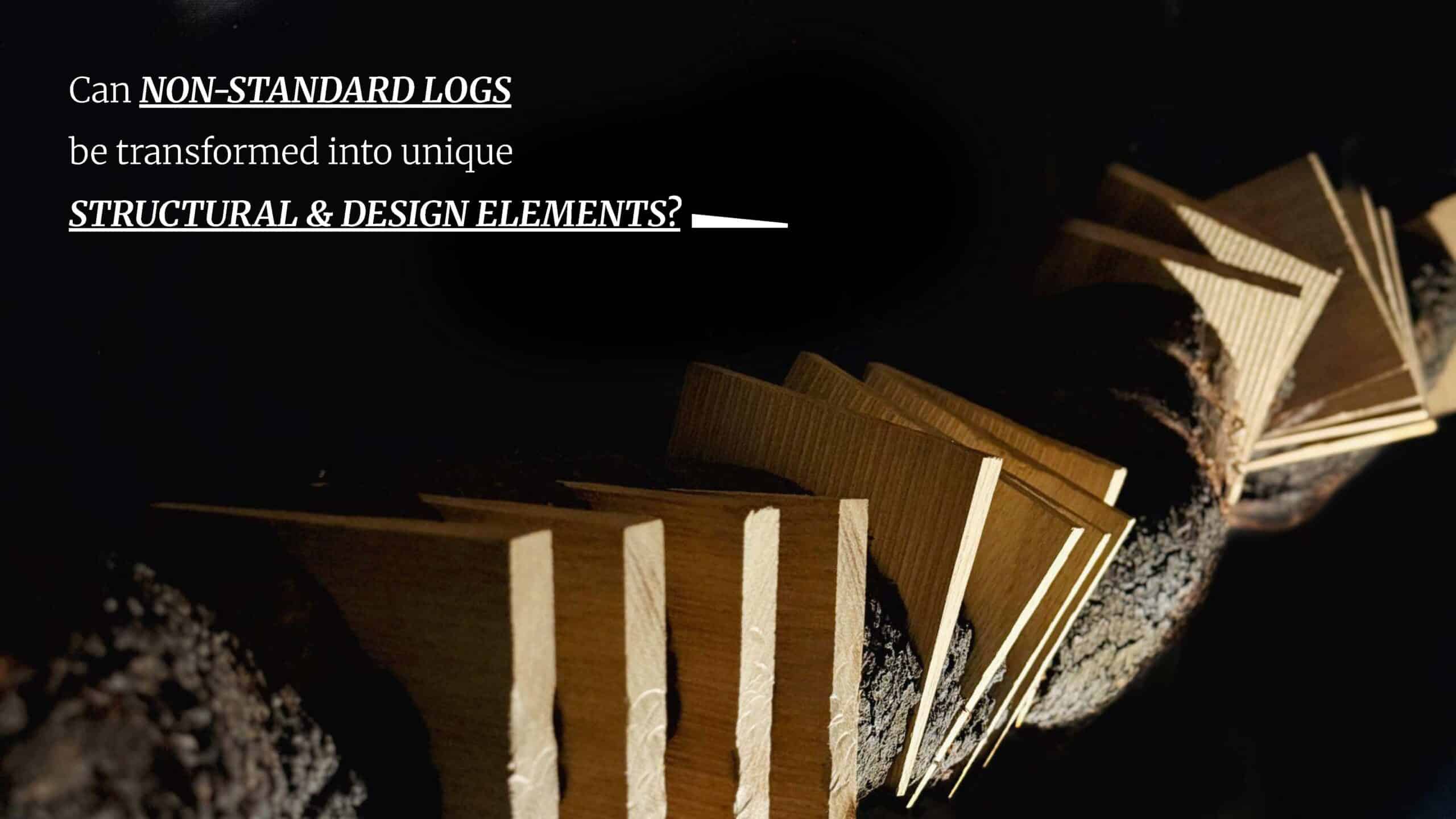
How? objectives and techniques
Our goal is to transform discarded logs into functional elements for structural and design applications through the use of advanced manufacturing technologies. By utilizing cutting-edge techniques, we aim to repurpose these materials in innovative ways, creating sustainable solutions that contribute to both architectural and industrial projects. This process not only helps reduce waste but also opens up new possibilities for the integration of natural materials into modern design.
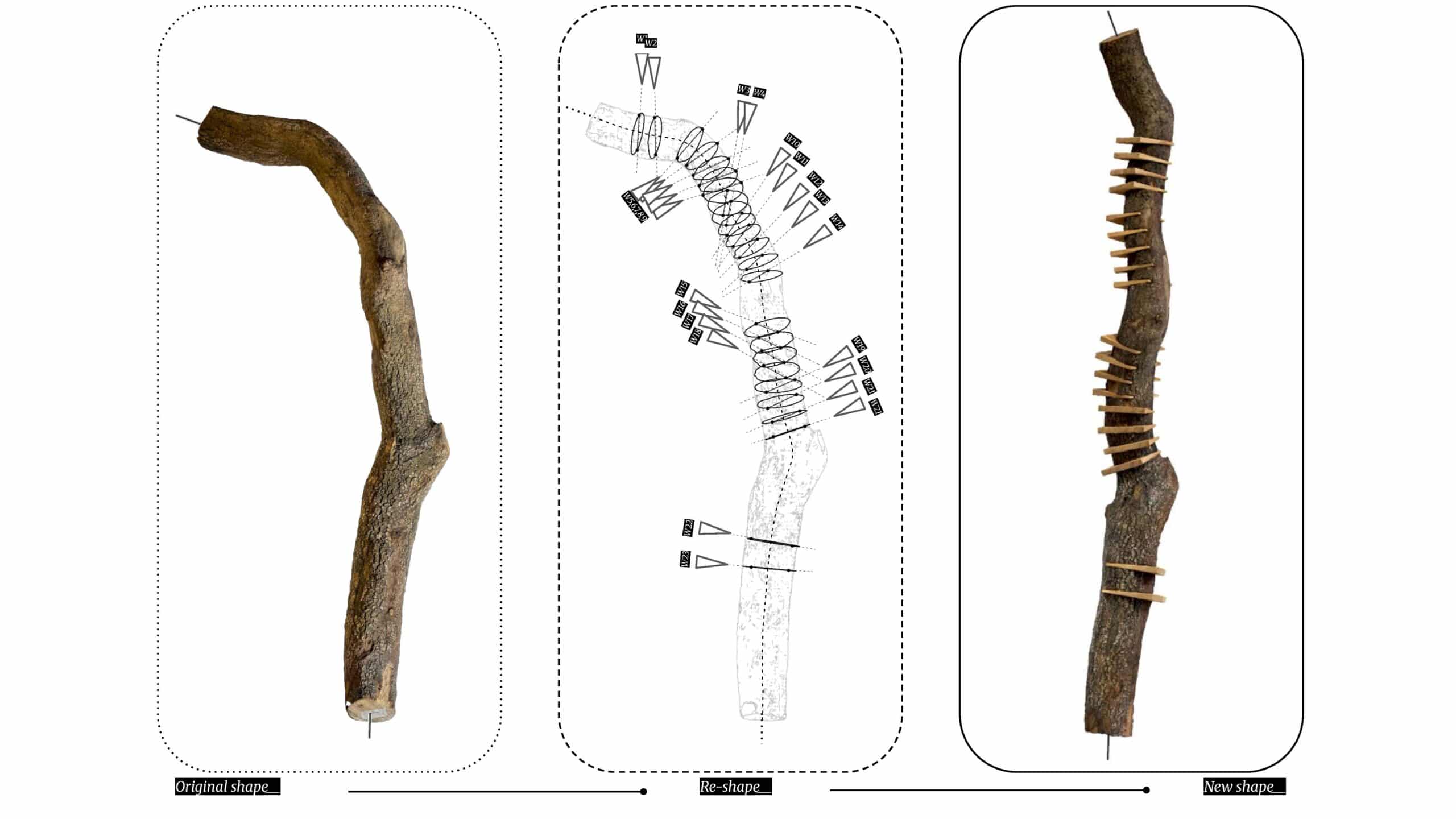
Kerfing
Kerfing is a woodworking technique that involves making a series of cuts, or “kerfs,” along the surface of the wood to allow it to bend and take on different shapes. This method is commonly used to create curves or complex forms that would be difficult to achieve with solid pieces of wood. While kerfing enables the wood to bend without breaking, it does weaken the material, reducing its overall structural strength. As a result, the wood may become more fragile, and the shape can be unstable unless additional reinforcement is added to ensure the integrity of the design.
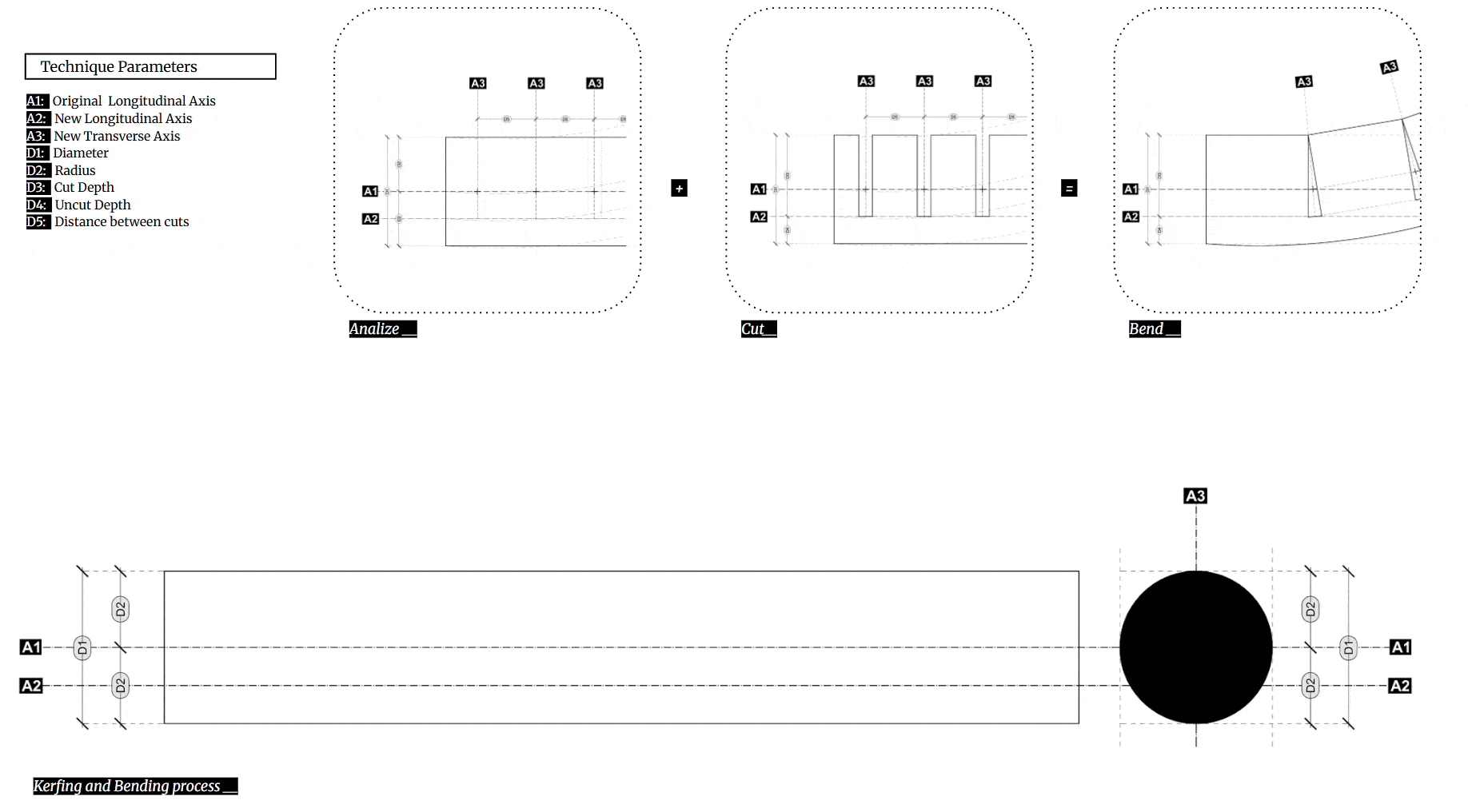
Kerfing is a woodworking technique that allows wood to be bent and shaped without the risk of breaking. This method involves making a series of cuts, or “kerfs,” along the wood’s surface to create flexibility and facilitate curved forms. It is commonly used in several fields, including:
- Musical Instruments: Kerfing is used in the construction of musical instruments, such as guitars and violins, where it helps shape the curved bodies of the instruments while maintaining their structural integrity.
- Furniture Design: In furniture design, kerfing allows designers to create curved or organic shapes in pieces like chairs, tables, or shelves, offering aesthetic appeal and functional flexibility.
- Nautical Architecture: Kerfing is also applied in nautical architecture, particularly in the construction of boat hulls, where it is essential for creating smooth, flowing curves that are both strong and lightweight.
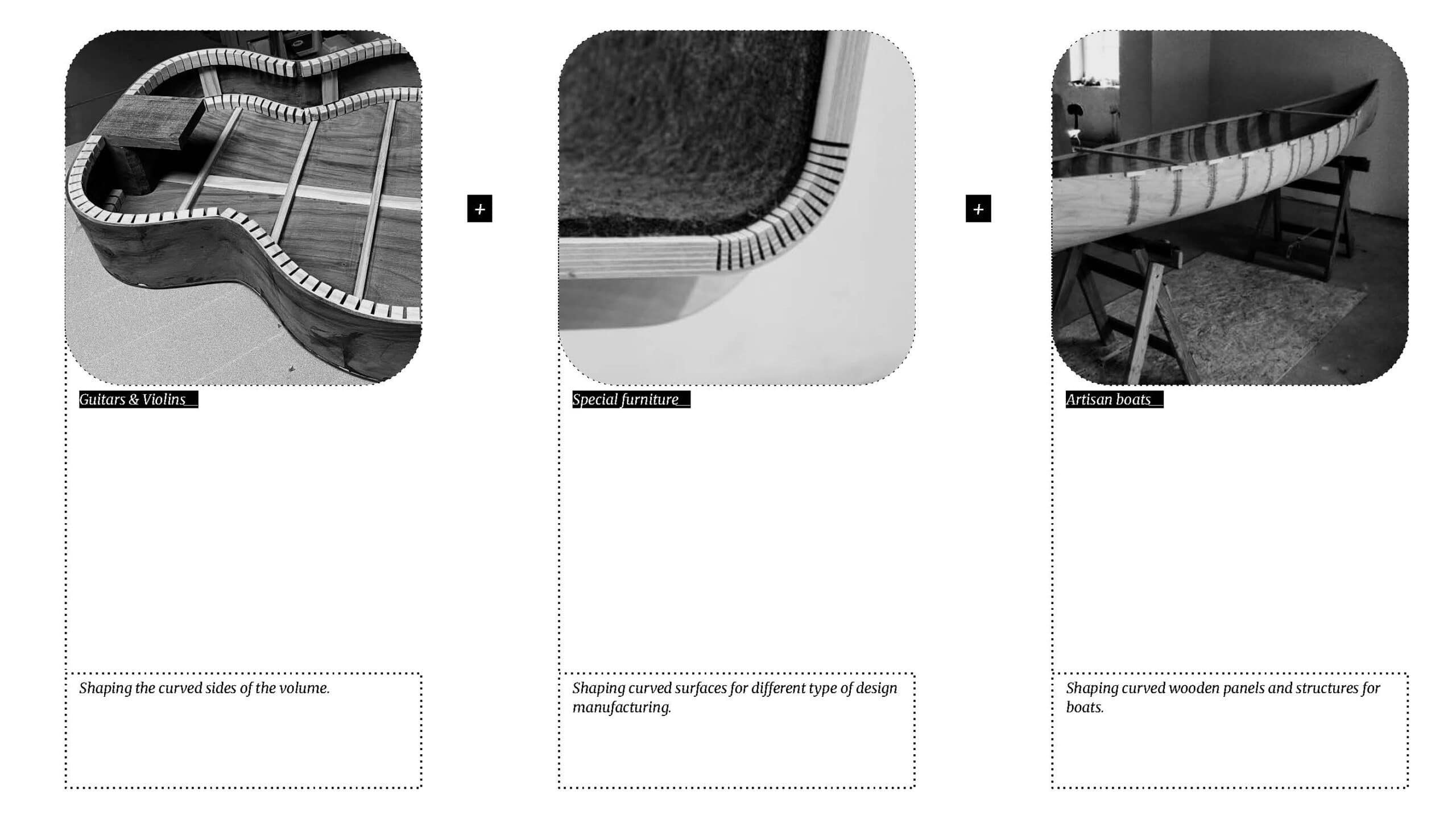
Our version of the technique
Wedge Kerfing is a variation of the traditional kerfing method that involves making cuts in the wood and then inserting wedges into these cuts to guide and enhance the bending process. This technique helps achieve more controlled and precise curves. Unlike traditional kerfing, which weakens the wood by creating gaps, wedge kerfing overcomes this structural weakness by adding material into the cuts, effectively improving the wood’s compression strength. The inserted wedges help stabilize the shape, making the curved wood more stable and structurally sound, even without additional reinforcement. This method is especially useful when stronger, more durable curves are needed for various applications in woodworking and design.
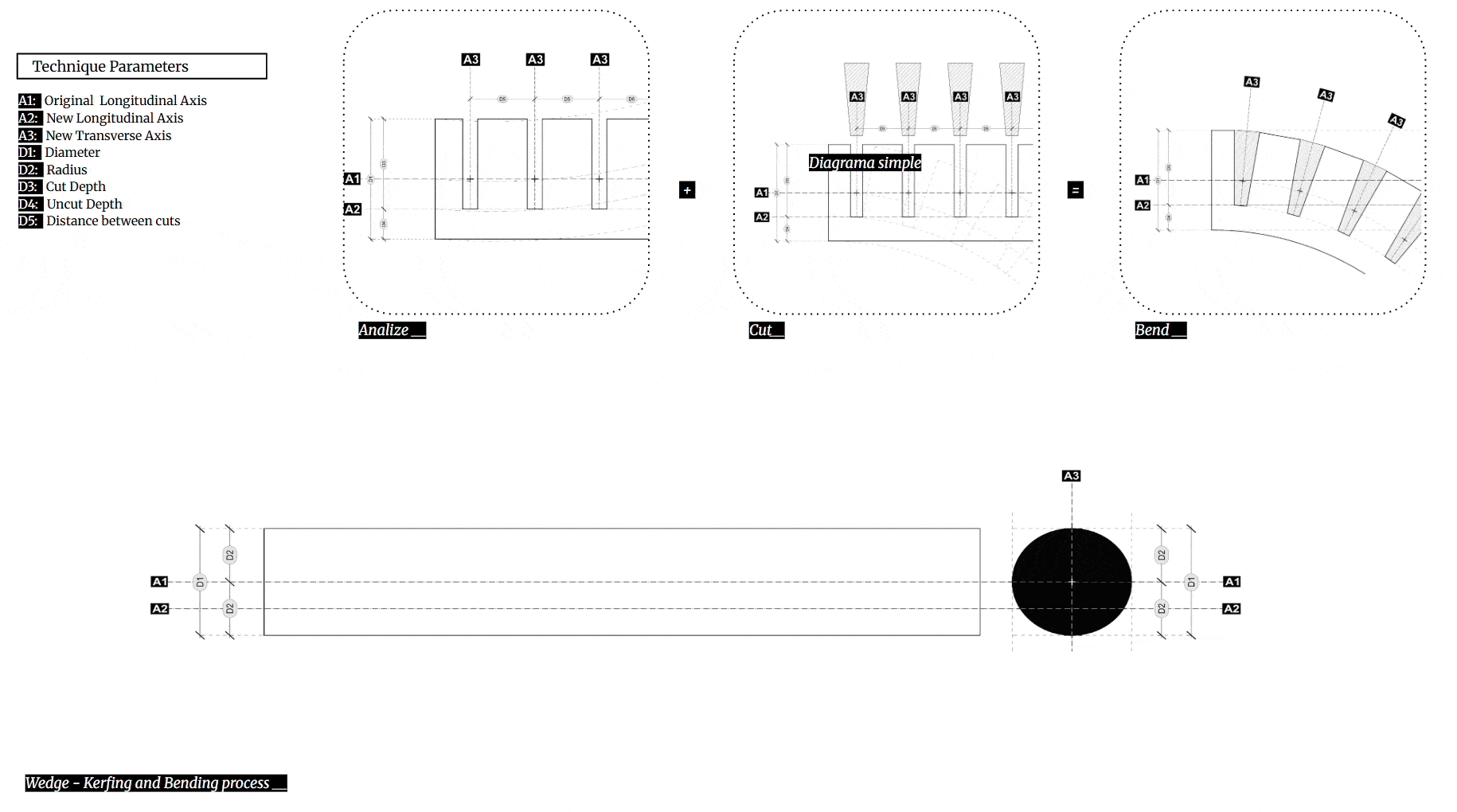
Material Limitation
Working with raw logs involves embracing irregular geometries, natural defects, and the unpredictable nature of each piece of wood. Unlike standardized lumber, raw logs require tools that can adapt to their unique shapes and conditions.
While table saws and CNC machines are well-suited for making precise cuts on uniform, standardized stock, they struggle when dealing with organic, non-standard materials. In contrast, industrial robots provide the flexibility, adaptability, and precision necessary to work with irregular timber. By scanning and responding to the unique 3D geometry of each log, robots can perform complex cuts and operations that would be impossible—or highly inefficient—using conventional tools. This makes them essential for advanced, adaptive manufacturing processes that involve raw, unprocessed wood.
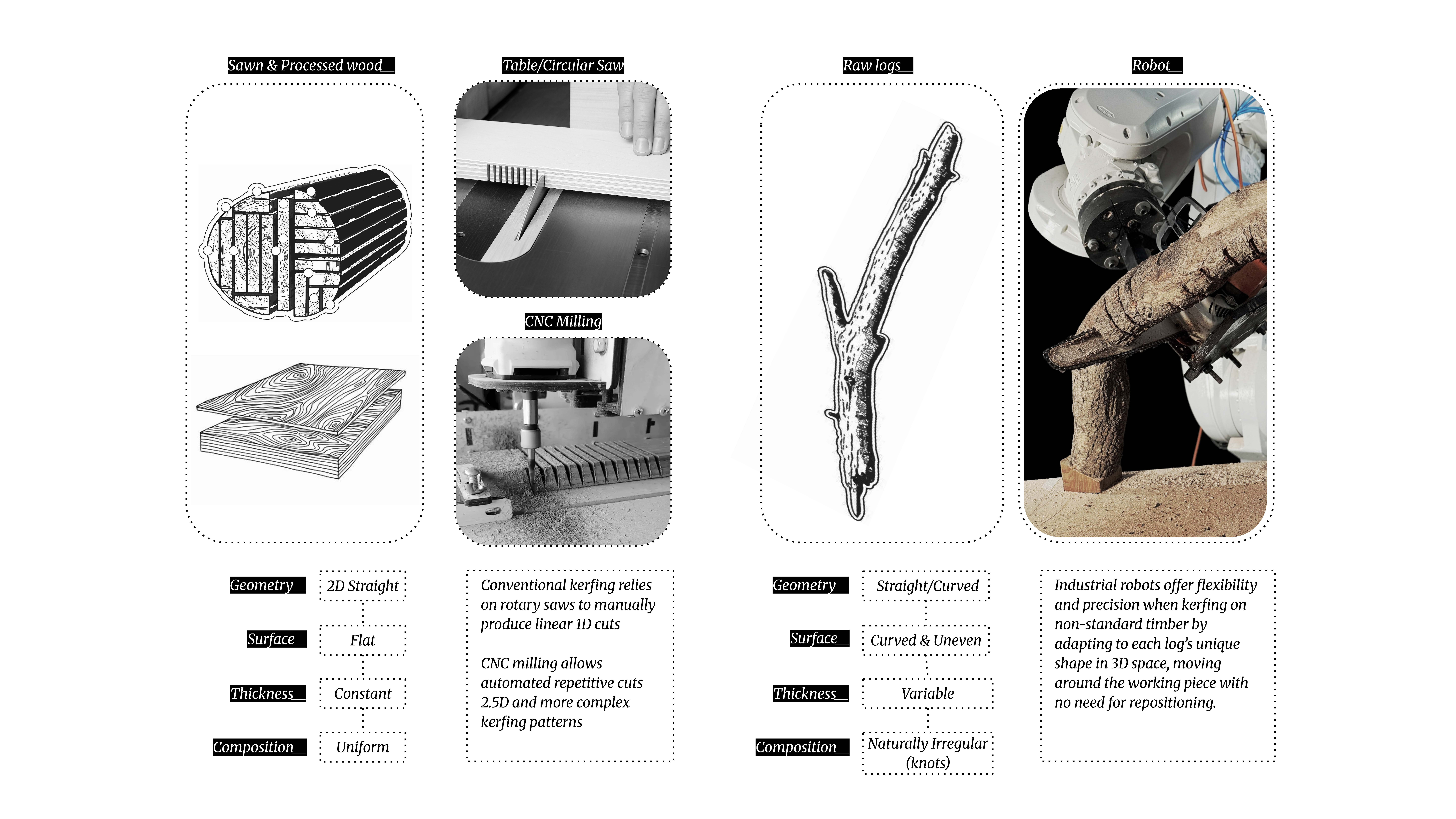
Prototypes
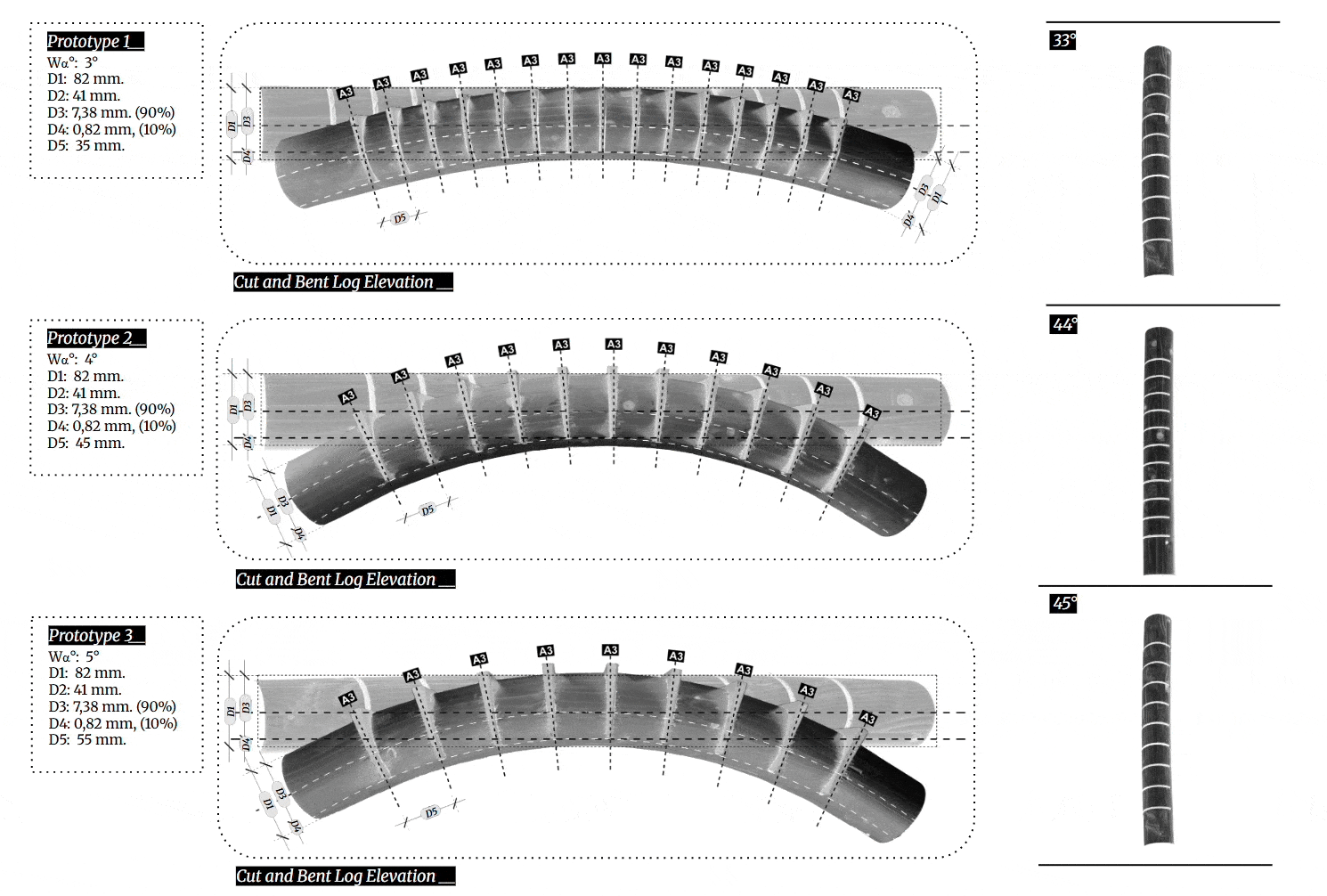
Workflow
Our workflow is a complete system to transform discarded logs into functional elements using advanced manufacturing technologies. It starts with evaluating, selecting, and scanning the logs. Then, we analyze each log digitally to define its internal axis, cutting planes, and the angles for the wedges. In the next step, we use robotic fabrication: the log is positioned, the cutting tool is prepared, and precise cuts are made, adapting to the unique shape of each log. Finally, we clean and soak the log, insert the wedges to guide and strengthen the curve, and finish with a stable product ready for structural or design applications.
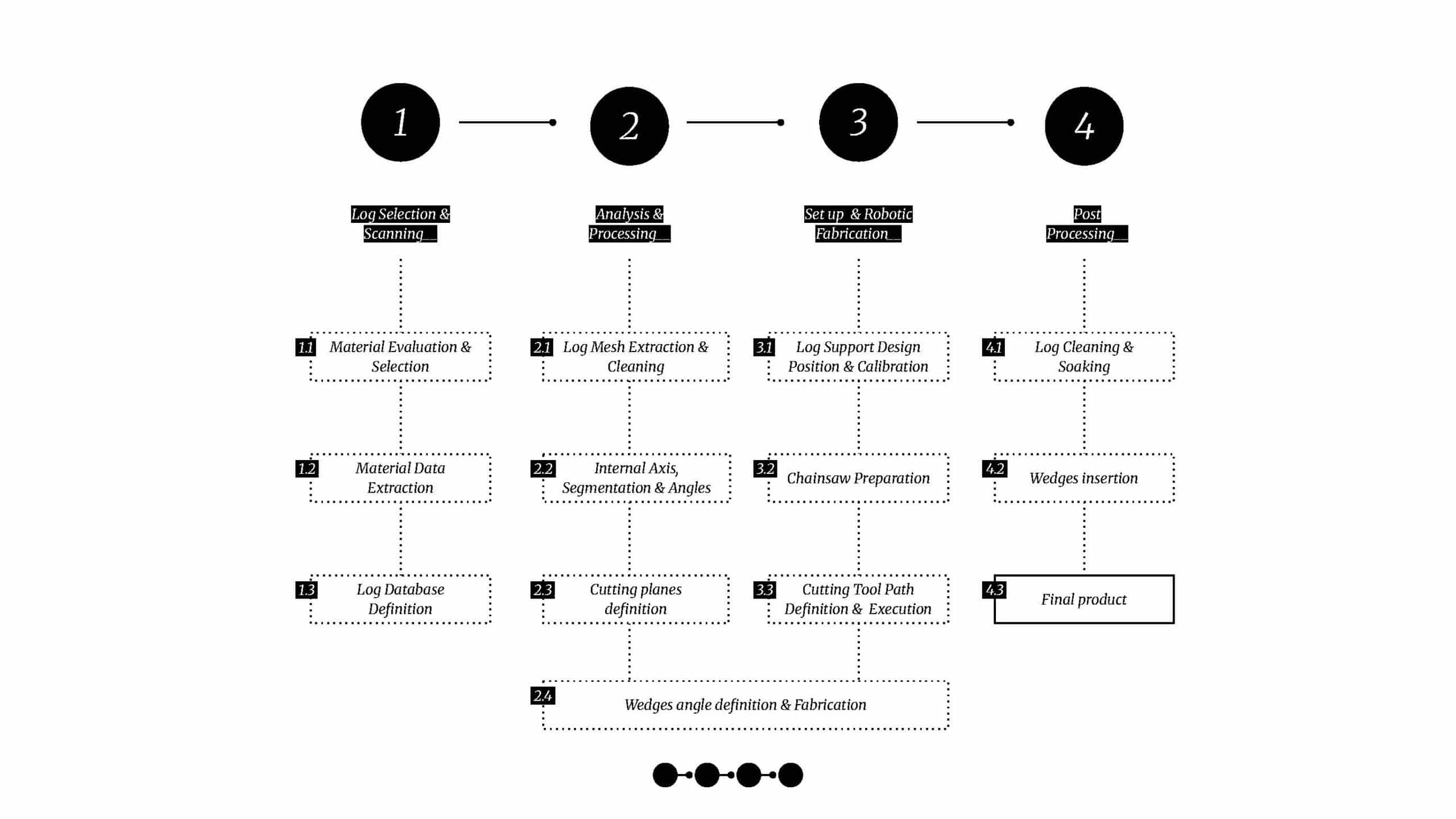
1.- Log selection / Log Scanning
Our process begins by selecting discarded logs to repurpose them into new functional applications. To understand the unique shape and structure of each log, we analyze their spatial geometry using 3D scanning technologies. This includes tools like the iPhone with Polycam and the real-time ZED camera, which allow us to capture accurate mesh data. All the scanned information is then organized into a log database, serving as the foundation for further design and fabrication steps.
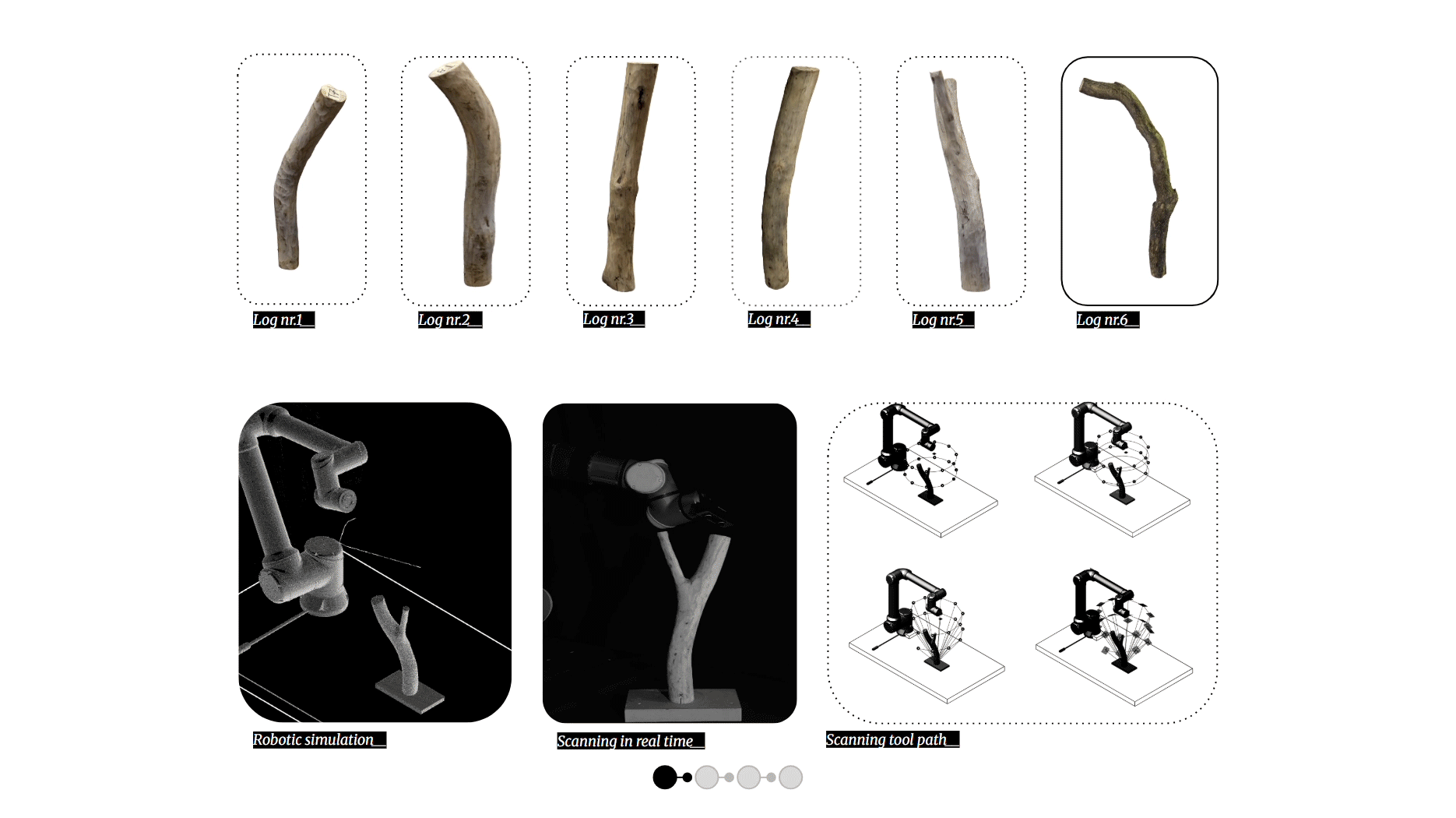
2.- Log Analysis / Desing Process
This slide focuses on the Log Analysis and Design Process stages of our workflow, which are essential for understanding and reshaping irregular timber. The goal of this phase is to identify the geometry, contours, and central axis of each log, and then develop a cutting strategy that allows for precise and efficient reshaping.
The process begins with mesh cleaning and the extraction of the internal axis (A1), which provides a reliable reference line for further operations. Next, we avoid knots and segment the log along its axis, dividing it into manageable parts that reflect its natural geometry. After segmentation, we analyze the angles of each section and assess how these will influence the final shape. Finally, we define the cutting planes and group them based on directional changes in the log’s shape (A1 & A2), ensuring that each cut supports the desired transformation.
This analytical approach allows us to treat each log as a unique object, respecting its organic form while preparing it for precision cutting and controlled bending in the next fabrication stages.
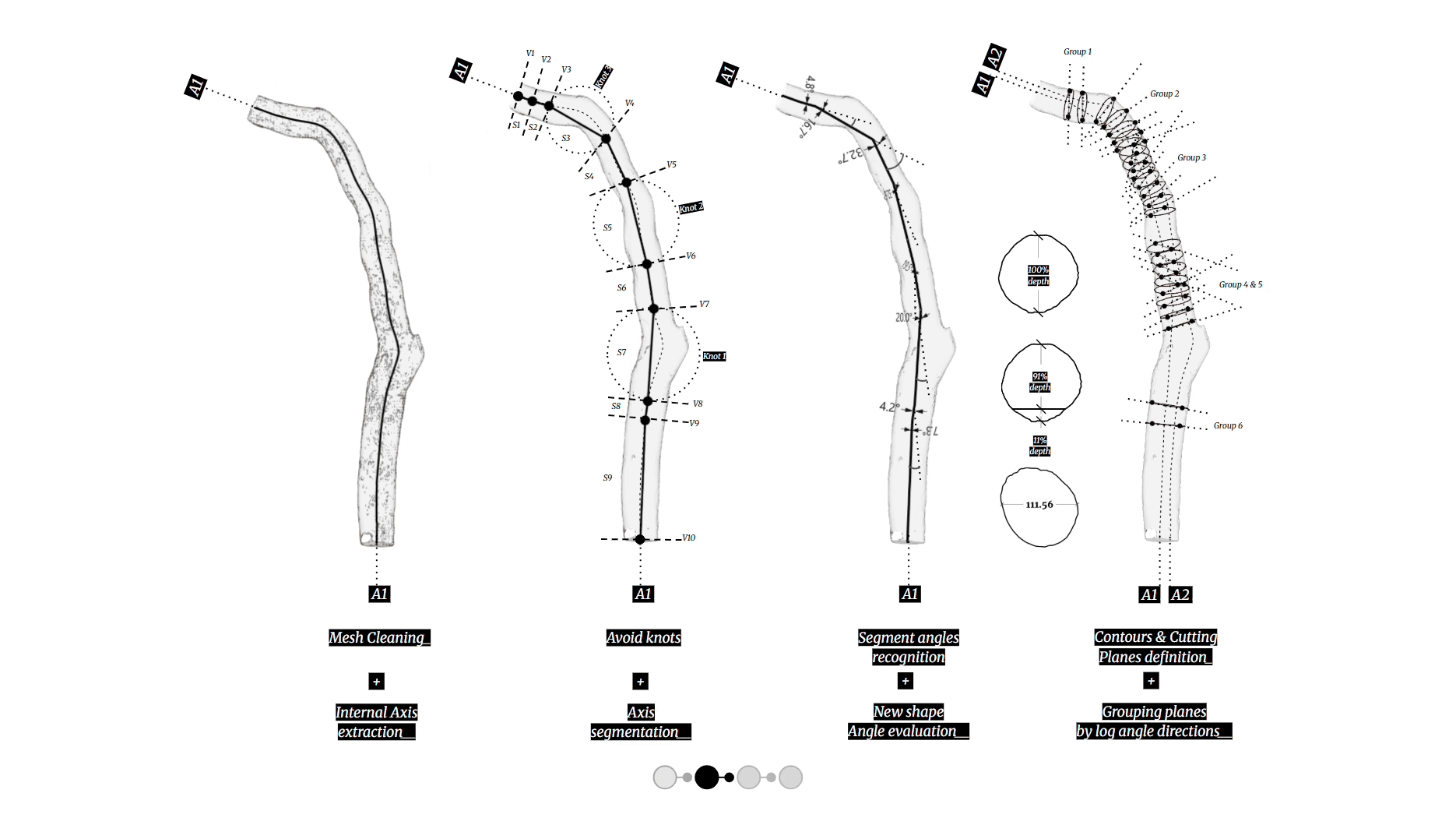
3.- Set up / Robotic Fabrication
This slide illustrates the Set Up and Robotic Fabrication stage of the workflow, where precision and coordination between machine and material are key.
Under Set Up, we focus on preparing and calibrating the position of both the cutting support and the tool. This involves scanning the log in place and adjusting its orientation on the support structure to align with the predefined cutting planes. The chainsaw is also calibrated — both in terms of position and angle — to ensure precise alignment with the log’s geometry.
In the Robotic Fabrication phase, we prepare the robot’s toolpath for cutting, based on the previously defined strategy. Key parameters include:
- Total cuts: 20
- Time per cut: 2 minutes
- Total cutting time: approximately 2 hours
- Initial cut speed: 10%
- Full cut speed: 15%
- Chainsaw oil refill: required after every 4 cuts
This phase is where all the digital analysis and planning translate into physical action, with the robot executing precise cuts on irregular logs using real-time calibration, ensuring consistency, safety, and control throughout the process.
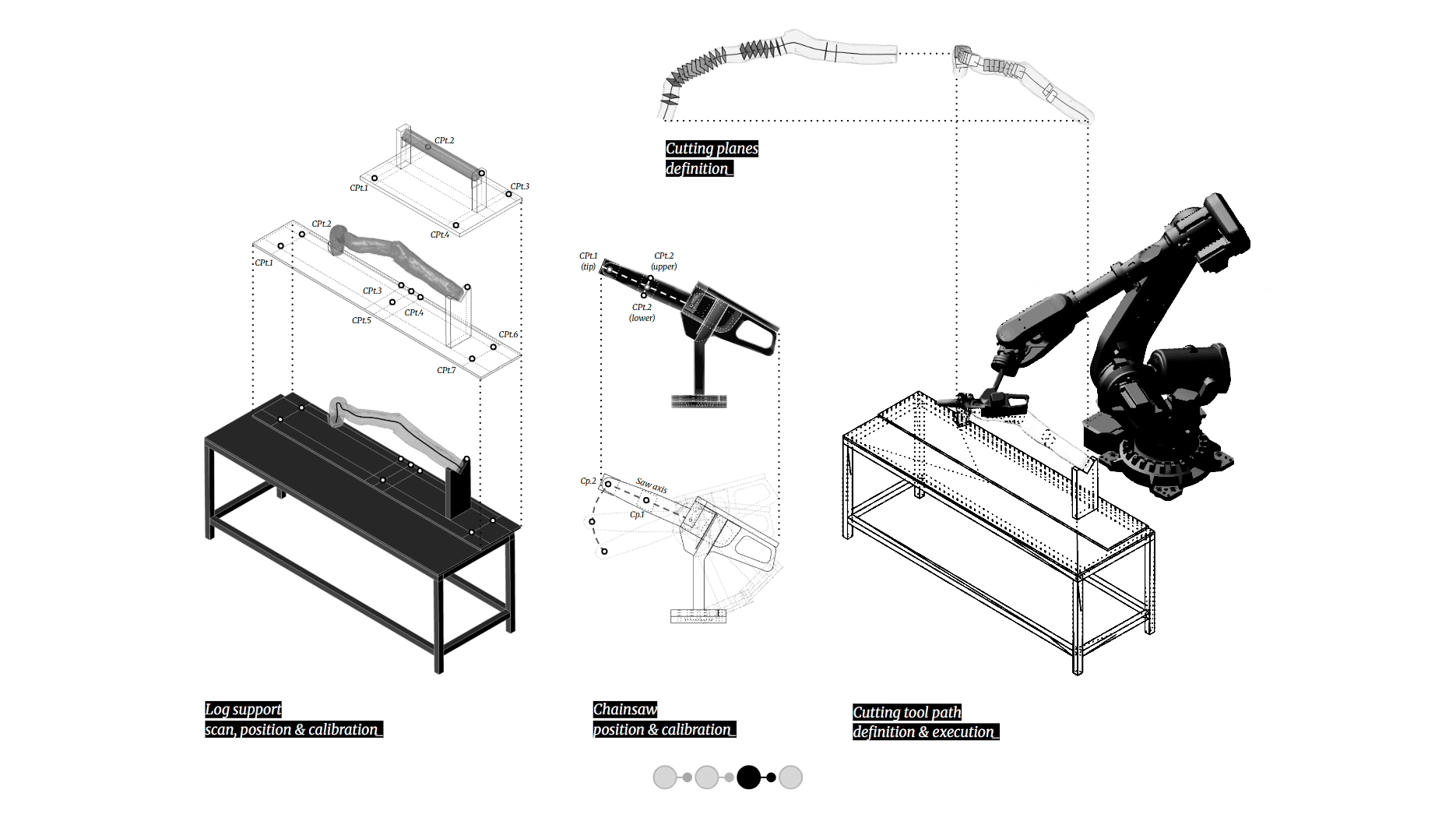
4.- Post Processing
In this stage, the kerfed log undergoes post-processing to enable and stabilize its new shape. First, the log is soaked in water, allowing the wood fibers to soften and become more flexible for bending. Once pliable, wooden wedges are manually inserted and glued into each kerf cut. These wedges help guide the curvature and maintain the new geometry by filling the gaps created during cutting. This process significantly reduces the log’s axis deviation and footprint, resulting in a more compact and structurally stable element, ready for further application in design or construction.
- The original log presents an irregular shape with an axis deviation of 70°, a height of 148 cm, and a footprint of 38×42 cm.
- In the kerfing stage, 20 cuts are made, each taking 2 minutes, resulting in a total cutting time of 40 minutes. The cuts reach a depth of 90%, with a kerf width of 5.5 mm and a wedge angle of 5°.
- For the bending process, the kerfed log is soaked in water, softening the fibers to allow for easier manipulation. Then, wooden wedges are manually inserted and glued into each kerf cut. This method reinforces the structure while guiding the new shape.
The result is a bent log with a significantly reduced axis deviation of 21°, increased height of 168 cm, and a more compact footprint of 22×22 cm—demonstrating the effectiveness of the method in reshaping and refining irregular timber for new design and structural purposes.
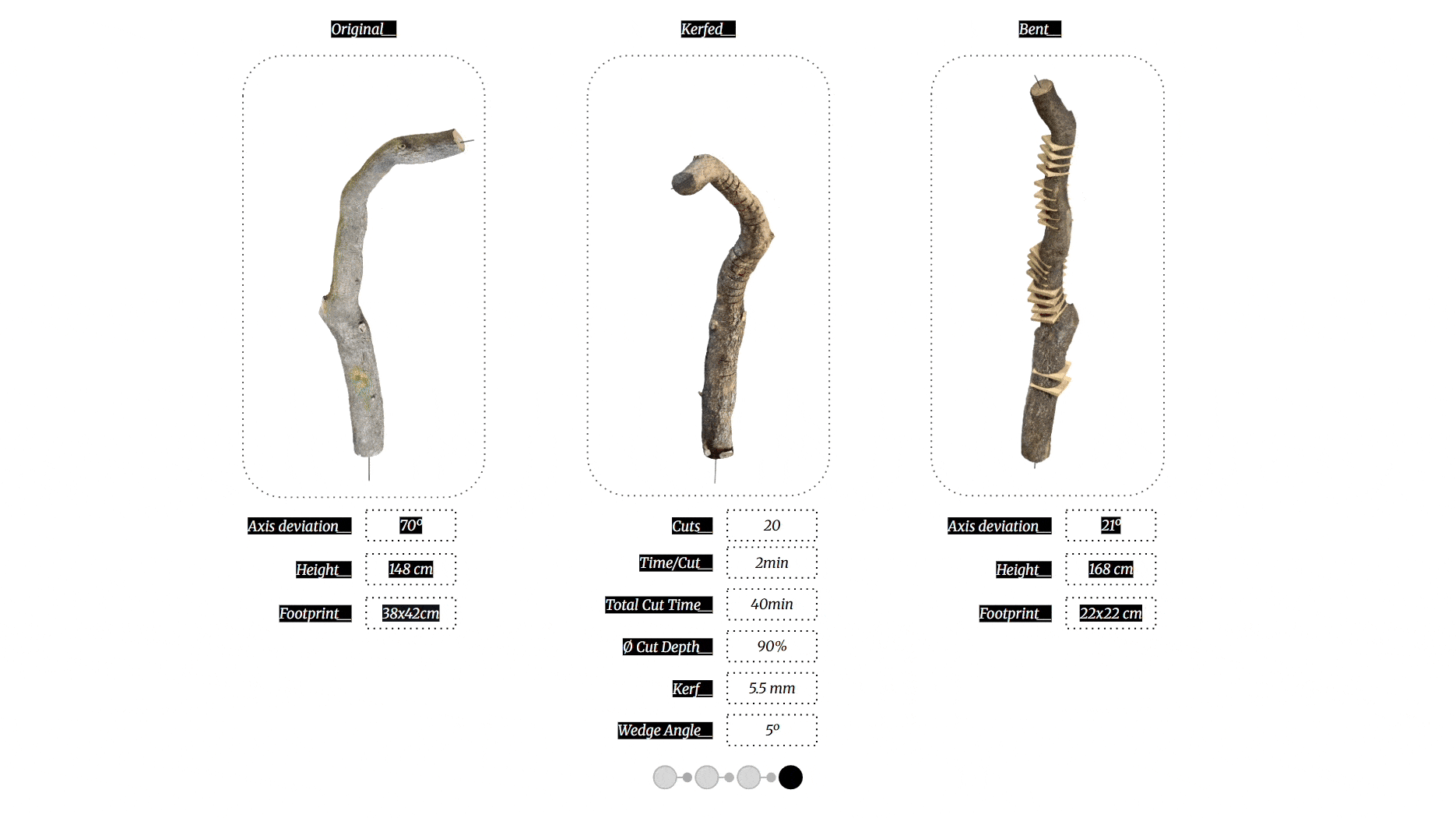
Current State
The current state of the project shows promising results in reshaping irregular logs through a controlled and replicable process. The system has been able to correct 70% of the original axis deviation, achieving a much straighter and more functional geometry. Additionally, the intervention results in a 12% increase in height, making better use of the material’s vertical potential, and a 48% reduction in footprint, allowing for more efficient spatial use and structural integration.
These improvements demonstrate the system’s effectiveness in compression-based applications. However, some limitations remain. The process depends on glue to handle tension forces, and natural wood irregularities—such as knots and forks—can interfere with kerf placement. The log’s diameter and length define the limits for wedge spacing and quantity. On the fabrication side, the robotic setup must be adapted to each unique log, and the chainsaw requires slow cutting speeds, continuous oiling, and maintenance. Finally, soaking the logs demands large containers, and each species of wood behaves differently depending on its elasticity (MOE) and resistance to failure (MOR). Despite these challenges, the project confirms the potential of this method for sustainable and high-precision reuse of discarded timber.
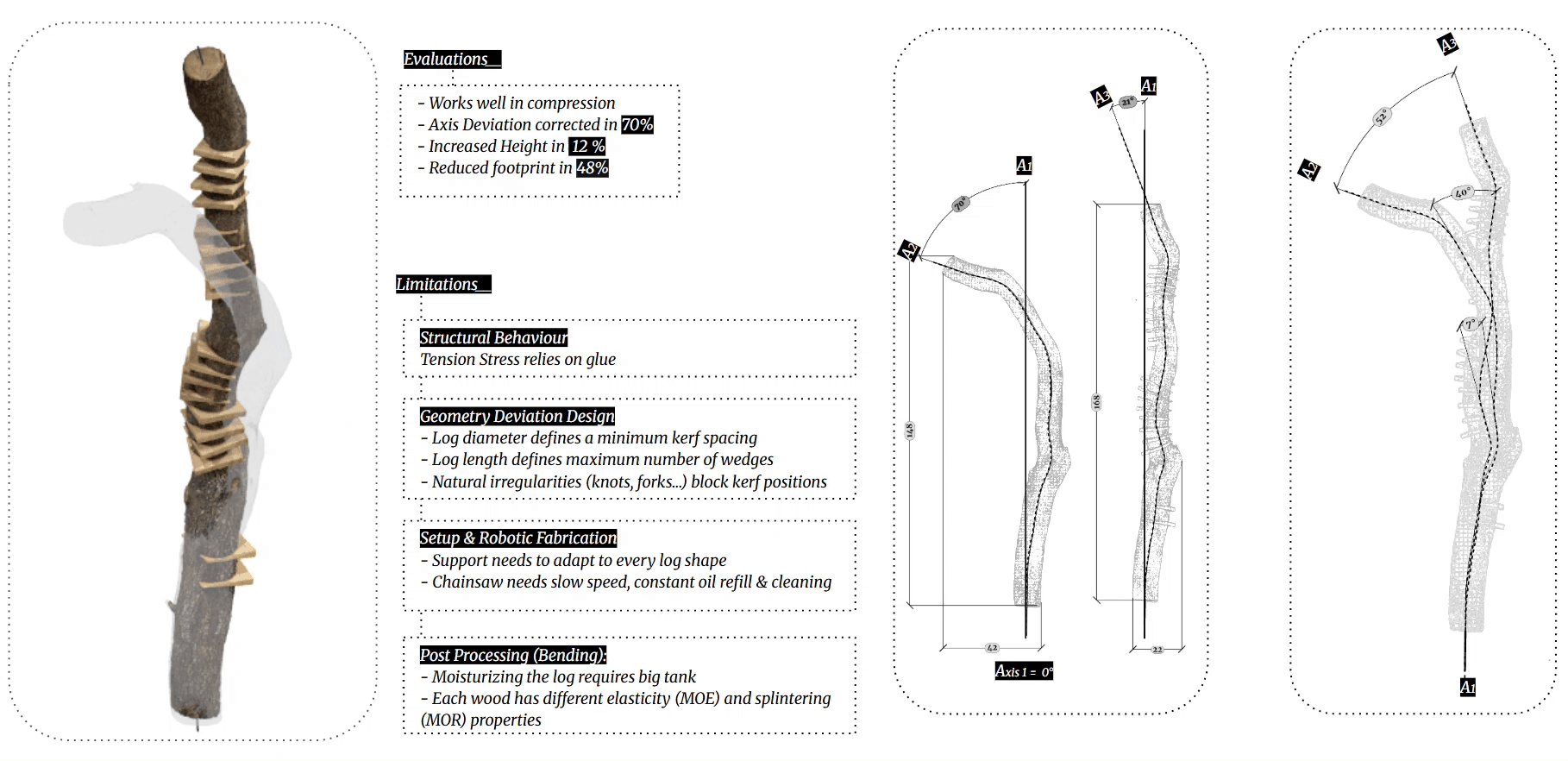
Application & Further Steps
The next steps of the project focus on improving performance, refining the process, and exploring new material and structural possibilities. On the structural side, upcoming tests will aim to develop mechanical solutions to manage tension stress, which is currently handled through glued wedges. These tests will inform the development of new structural strategies for more stable and reliable outcomes.
In terms of geometry design, the workflow will be optimized to better avoid natural wood defects such as knots and forks, and to allow for more efficient and controlled bending. For robotic fabrication, the team will develop adaptable supports that can adjust to different log geometries and explore vertical log positioning to improve robotic reach and precision.
During post-processing, the focus will be on testing more elastic wood species like chestnut, beech, or ash, which may allow for smoother bending and fewer failures during deformation.
As for applications, once structural testing is complete, the project aims to propose viable architectural uses. Given that the system already performs well in compression, the most promising uses include columns, arches, and compressed elements in truss systems, where the reshaped wood can be integrated efficiently and meaningfully.
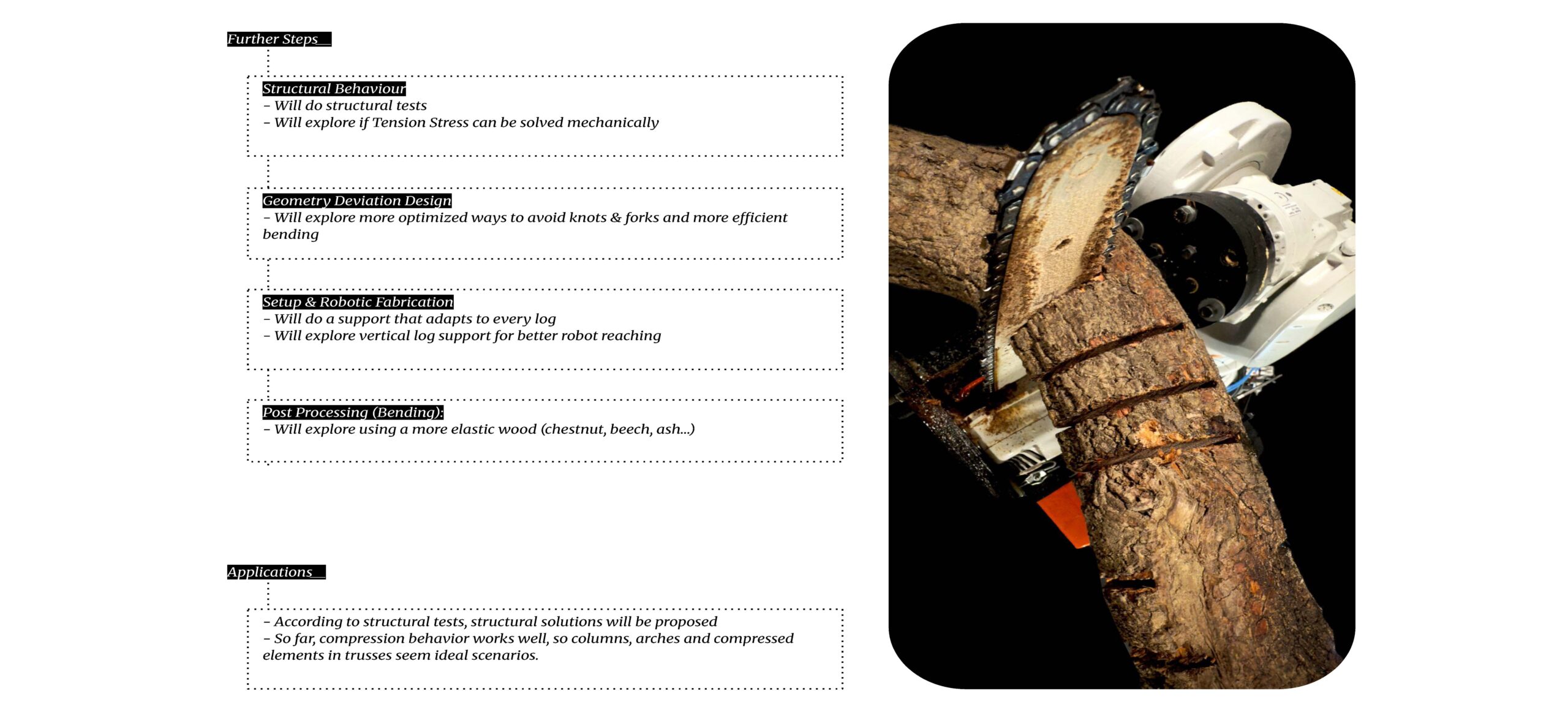
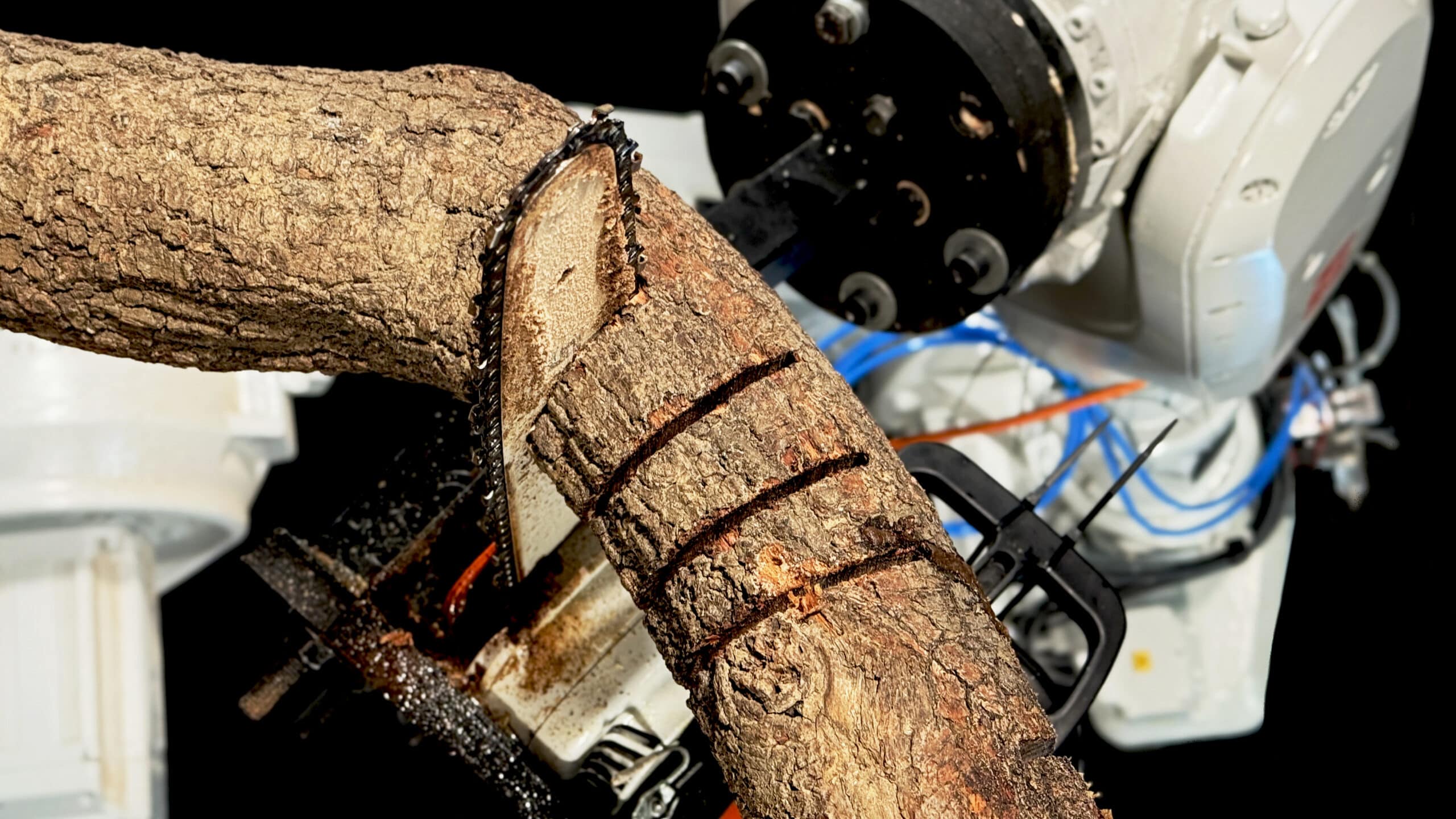
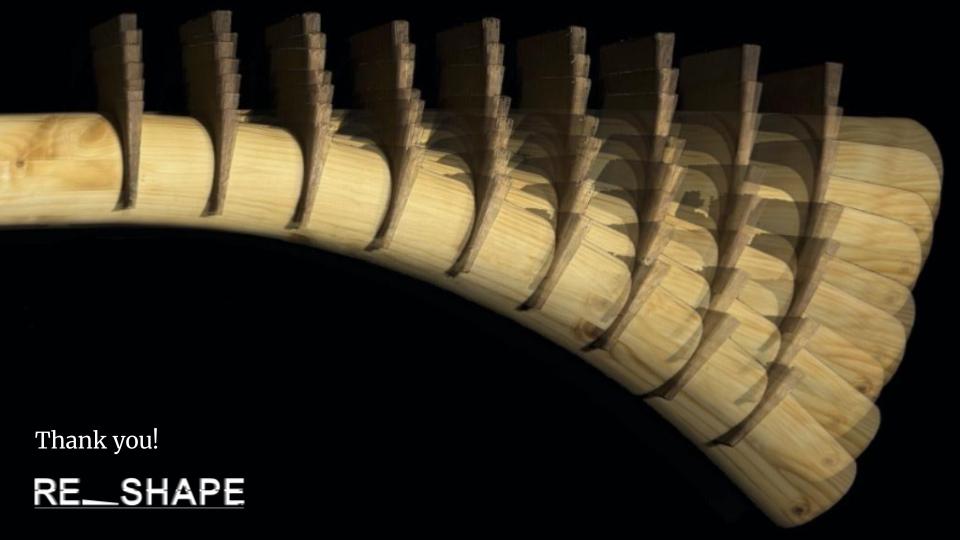